The unresolved ecological and evolutionary role of fungal fruit body coloration
- 1Fungal Ecology and BayCEER, University of Bayreuth, Bayreuth, Germany
- 2Department of Climate Change Impacts on Agroecosystems, Global Change Research Institute of the Czech Academy of Sciences, Brno, Czechia
Fruit body-forming fungi are hyperdiverse and of central importance for the functioning of ecosystems worldwide. They provide habitat and resources for other organisms and perform critical roles in carbon and nutrient cycling. Like in animals and plants, fungal coloration is expected to play a fundamental role in response to biotic and abiotic environments, thus providing invaluable information to predict fungal and associated diversity in the Anthropocene. Despite centuries of plant and animal coloration research, the role of fruit body colors in fungal ecology remains mostly obscure. Essential questions are unresolved, such as: How do fruit body colors function to cope with abiotic stress? Do fruit body colors function to attract dispersal vectors or prevent predation via camouflage or aposematism? What is the significance of fruit body colors for fungal fitness? What are the implications of climate change-induced fruit body color change on fungal and associated biodiversity? Here, I review existing knowledge and outline several research trajectories to better understand the ecological role of fruit body colors. Revealing climate-driven adaptations and interactions with other organisms will improve forecasts under climate change for fungal diversity and interrelated biodiversity and inform cross-taxonomic conservation strategies.
Introduction
Fungi constitute one of the largest groups of organisms on Earth (Niskanen et al., 2023). They are crucial in carbon and nutrient cycling, providing ecosystem stability (Liu et al., 2022). Fruit body-forming fungi have evolved unique decay abilities (Floudas et al., 2012) as well as plant symbioses (Kohler et al., 2015) and provide resources to other organisms for breeding (Lunde et al., 2022) or nutrition (Fogel and Trappe, 1978; Elliott et al., 2022; Kitabayashi et al., 2022). Gaining a mechanistic understanding of the biotic and abiotic drivers of biodiversity is fundamental to forecasting and predicting fungal species and community performance and associated diversity in climate change (Urban et al., 2016). Forecasts of biodiversity have implications for the management of protected areas (Hannah, 2010), conservation strategies (Dawson et al., 2011), and policymaking (Watson et al., 2019). Functional traits and experiments allow for a mechanistic understanding of species’ adaptations to their environment and thus provide a better basis for forecasts than species diversity measures (McGill et al., 2006; Urban et al., 2016). Coloration, one of the most important functional traits on earth (Cuthill et al., 2017), is a complex trait with multifunctional roles as a visual signal and as pigments. Biotic processes include attraction, camouflage, aposematism, mimicry (Chittka and Raine, 2006), microbial defense (Narsing Rao et al., 2017), and dispersal (Lomáscolo et al., 2010). Abiotic processes include protection from abiotic stress such as extreme temperatures via thermoregulation (Trullas et al., 2007; Delhey, 2019), UV radiation, desiccation, physical damage, or heavy metals (Gill and Tuteja, 2010; Vavricka et al., 2010; Cordero and Casadevall, 2017). However, despite the enormous research on color in animals and plants (Chittka and Menzel, 1992; Caro, 2017; Cuthill et al., 2017), we lack even the most fundamental understanding of the multifunctional ecological roles of fruit body colors and, therefore, knowledge of the consequences of climate change for fungi and the biodiversity depending on fruit body formation. Fruit bodies are the organ of sexual reproduction of ca. 26,000 fungal species only within the Agaricomycetes (Sánchez-García et al., 2020). Their colors are important features in the systematic research (Guarro et al., 1999), species identification (Læssøe and Petersen, 2019), and the industry (Afroz Toma et al., 2023).
Pigments and colors in fruit bodies
Pigments found in fruit bodies are mainly melanins (dark brown), carotenoids (orange, yellow, and red), and betalains (yellow, red, and violet) (Gill, 1999; Velíšek and Cejpek, 2011). Fruit body colors are mainly brownish or whitish, but many fruit bodies are also brightly red, yellow, purple, or greenish (Figure 1A). These colors are, however, based on human vision color models and do not capture what other organisms might interpret. Currently, there are no studies of fruit body reflectance spectra (except some single species) (Bekiaris et al., 2020), and therefore, a respective database is missing, which also restricts the translation of fruit body reflectance into different color vision models, e.g., of beetles and mammals. Furthermore, it is currently largely unclear how frequently fruit bodies reflect in the ultraviolet range. Although structural colors seem to be absent (Brodie et al., 2021), fluorescence appears to play some role (Reinhold, 2020). Thus, a critical step will be to generate a fruit body reflectance database and use it to calculate textual colors for different organism visual models.
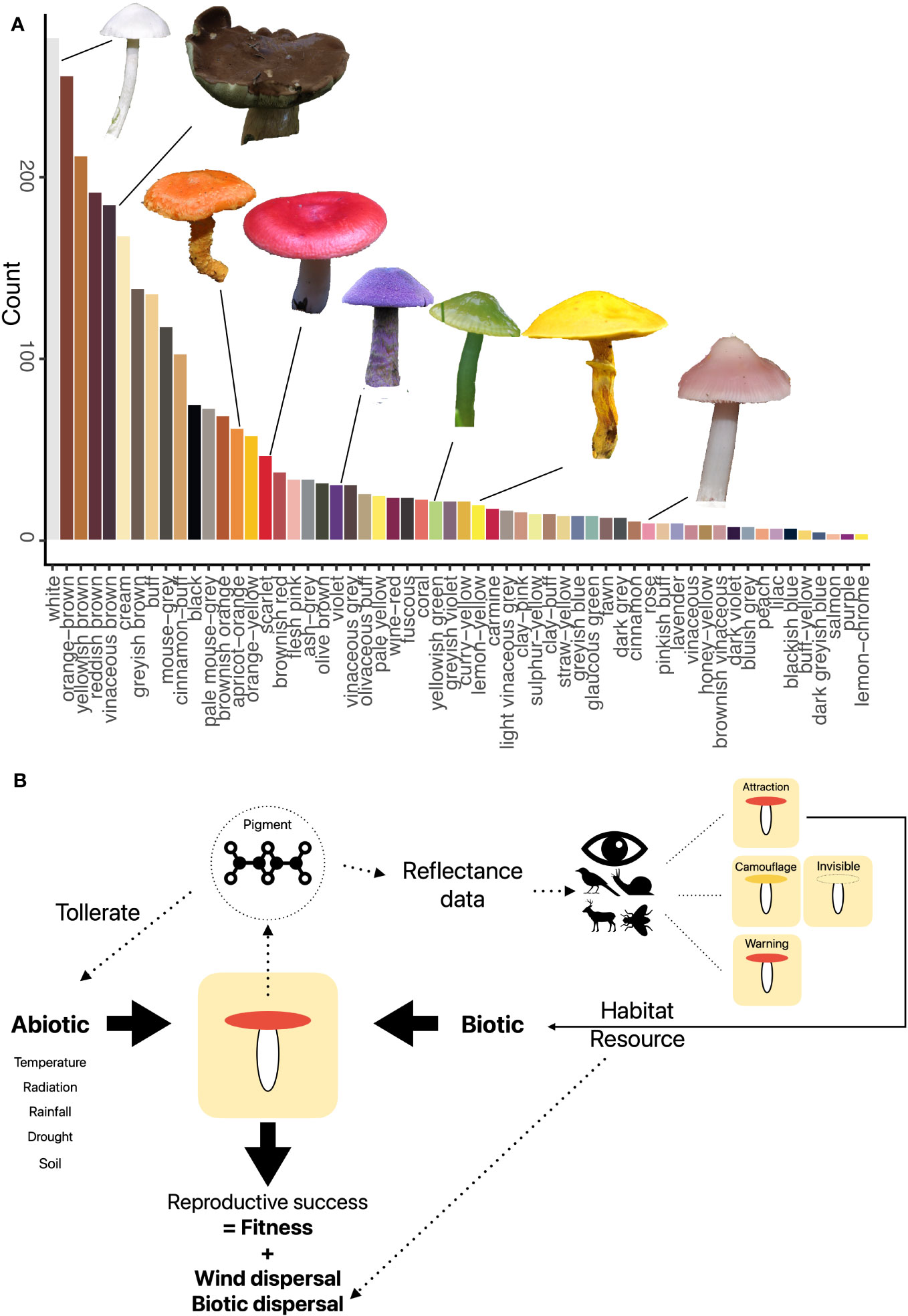
Figure 1 (A) Fruit body colors of 1,575 temperate fungal species based on a standardized color palette (Læssøe & Petersen, 2019). Fruit body images by Franz Krah. (B) Conceptual framework of the abiotic and biotic aspects of potential relevancy for the role of fruit body colors. Pigment production is driven by pigments, which also mediate responses to abiotic stress. Depending on the visual system, different colors are seen, which may lead to different animal behavior such as predation. Both abiotic and biotic factors may effect fungal fitness if they suppress maturation and spore release.
The diversity of colors is high among species; however, it may also be high among populations. Currently, we have generally only little information about the role of intraspecific trait variability in fungi (Krah and Bässler, 2021). Field identification guides often list the occurrence of varieties within species, which often differ in color (e.g., Knudsen and Vesterholt, 2008). There is currently no systematic assessment of color variability within species; however, a recent publication found that the high color diversity in Boletus edulis could be partly explained by the population structure (Tremble et al., 2023). Thus, when fruit body reflectances are measured, the variability within the species should ideally be captured.
Biotic and abiotic factors
Both biotic and abiotic environmental factors relate to the coloration of organisms (Figure 1B).
Biotic factors
Within animal and plant studies, biotic mechanisms include attraction (e.g., pollinators, dispersers, and mating partners), aposematism and mimicry (e.g., repellence of consumers), camouflage (e.g., avoidance of consumers) (e.g., outlined in Caro, 2017), and antimicrobial activities (Cordero and Casadevall, 2017). First, fungus–animal interactions are frequent, including the utilization of fruit bodies as a food source by mammals and slugs, as a breeding ground for beetles and flies, or simply as a resting site for flying insects (Elliott et al., 2022; Borgmann-Winter et al., 2023). Attraction might play a role in dispersing sexual spores produced in the fruit body. Many studies have indeed suggested that animals may contribute to spore dispersal likely on small spatial scales (Guevara and Dirzo, 1999; Lilleskov and Bruns, 2005; Seibold et al., 2019; Elliott et al., 2022; Borgmann-Winter et al., 2023); however, wind dispersal is relevant for both short- and long-distance dispersal (Finlay and Clarke, 1999; Hallenberg and Kuffer, 2001; Galante et al., 2011; Golan and Pringle, 2017). Furthermore, the attraction could also signal profitability, similar to the color change of plant fruits when ripe (Sinnott-Armstrong et al., 2021). Although studies have shown that the nutritional value of fruit bodies varies with their developmental stages (Cheung, 2013), we do not know if, e.g., dietary fiber content correlates with color changes. Finally, fruit body color has been suggested as a warning (aposematism) of their unprofitability or poisoning to herbivores. First, we currently need more observational data on repellent tastes and toxic properties (but see below on antimicrobial defense) and only some reports and speculations for single species (Halbwachs et al., 2016). Second, textual color categories based on human color sensitivity did not correlate with human-coded unprofitability (Sherratt et al., 2005). The latter paper found a strong correlation between color and spore size, indicating a role of color in dispersal by insects or mammals. Thus, the correlation between spore size and fruit body color should be examined in more detail. The paper further suggested relations between smell and poisoning, highlighting the need to investigate olfactory clues when studying fungal colors. These results highlight that we need to understand whether fruit body color provides biotic signaling leading to increased or decreased animal consumption or visitations.
Although attraction is important in plant–pollinator interactions, other biotic factors may play a role. Based on the human color model, ca. more than 50% of fruit body colors are somewhat dull colored (Figure 1A), maybe resembling forest floors, especially in fall, when most species produce fruit bodies in the northern hemisphere (Krah et al., 2023). Visual camouflage could deceive mycophores and protect fruit bodies by preventing detection (crypsis) against the background or their substrates or environments (Stevens and Merilaita, 2009). The matching of fruit bodies with their backgrounds may thus prevent detection. Since the key function of the fruit body is protecting spore production until the release of spores, camouflage could protect the fruit body long enough to avoid being consumed before spore discharge (Halbwachs et al., 2016; Nagy et al., 2017). However, the camouflage hypothesis has yet to be tested. Crypsis became known first in plants, where ca. 25% of all species are estimated to be cryptic or aposematic (Lev-Yadun, 2016). In fact, the lines between crypsis and aposematism are often not clear and many fruit bodies may be camouflaged and poisonous at the same time. In summary, we lack evidence in favor of or against the attraction and camouflage hypotheses.
Besides biotic factors based on visual signals, pigments may also play essential roles in the defense from bacterial and fungal attacks. Those may include aspects of aposematism or the killing of pathogens and predators. Chemical defense strategies have been reviewed elsewhere (e.g., Spiteller, 2015). For example, the pigment mycenaaurin produced by the orange-colored species Mycena aurantiomarginata exhibits antibacterial activity (Jaeger and Spiteller, 2010); however, the ecological significance is still unclear. Another largely unexplored topic is photoactivated defense strategies. Many basidiomycete pigments show structural similarity with plant pigments, which are known for light-activated defense strategies such as the production of radical oxygen species (Siewert, 2021). A first case study showed that some pigments of species of Cortinarius subgenus Dermocybe are photoactivated and had toxic effects on a glassworm species (Hannecker et al., 2023).
Abiotic factors
Besides biotic factors, the abiotic environment also interacts with color, specifically pigments. Pigments have been described for some species (Gill, 1999), primarily for food and medicinal (Kalra et al., 2020) or for taxonomic and systematic purposes (e.g., Lodge et al., 2014). However, we need pigment composition data for most species (but see Siewert, 2021).
One of the most studied abiotic aspects of color is thermoregulation. The thermal-melanism hypothesis for ectotherm organisms, such as fungi, states that dark-colored organisms are at an advantage in colder environments (Trullas et al., 2007). Using the first large-scale fruit body distribution dataset, colleagues and I found that fungal assemblages had, on average, darker fruit bodies in colder environments, supporting the thermal-melanism hypothesis for the fruit body-forming fungi (Krah et al., 2019). In subsequent studies, this finding was confirmed along with an elevational gradient and independent from the microclimate (Krah et al., 2022; Oechler and Krah, 2022). Further hypotheses regarding fruit body coloration are the desiccation-protection hypothesis (DPH; Oechler and Krah, 2022), which predicts color adaptations of organisms in dry environments, and the photo-protection hypothesis (PPH; Rensch, 1929), which predicts color adaptations of organisms in radiation-rich environments. Considering multiple covariates, Krah et al. (2019) found no support that darker colors are related to radiation protection but that assemblage color lightness varied with precipitation, maybe due to desiccation protection via pigments (Cordero and Casadevall, 2017). Despite these first vital insights, we still need to find out whether fungi respond plastically in terms of fruit body pigmentation to environmental factors, whether pigmentation can reduce environmental stress, and whether pigmentation provides a fitness benefit in specific conditions such as biomass or number of viable spores. Thus, additional studies relating measured color spectra and pigment composition to abiotic factors and testing for fitness advantage under controlled conditions are needed.
Besides ecological studies, phylogenetic comparative methods also provide important insights into the ecology and evolution of coloration. For example, plant species likely diversified in co-evolution with pollinating insects (van der Niet et al., 2014). In Agaricomycetes, only low phylogenetic signal of color lightness was found across ca. 3,000 species, suggesting rather adaptive evolution of pigments and colors (Krah et al., 2019). However, the low phylogenetic signal may also have resulted from considering only one aspect of color in terms of color lightness.
Future perspective
The summary of studies clearly shows a need for more research on the role of fruit body coloration in fungal ecology. Thus, in the following, I will highlight essential ways forward toward research trajectories required to fill the apparent research gaps. Those will include generating novel data and combining them with existing data to address macroecological questions. Furthermore, laboratory and field experiments are needed to test for abiotic effects on color and explicitly test for attraction/aposematism vs. camouflage. These studies should be complemented by functional and physiological studies focusing on how color mediates fungal responses to their environment and fitness.
Database and color models
The current greatest obstacle in studying the role of color in fruit bodies is the need for more data on fruit body reflectance spectra. Thus, research efforts should increase to measure reflectance. Furthermore, reflectance should be translated into different color vision models. Insects, mammals, birds, and slugs are relevant for fungi–animal interactions (Elliott et al., 2022). For instance, most mammals have two photoreceptors sensitive to green and blue light (Jacobs, 2009); most insects have either three or four photoreceptors sensitive to UV, blue, and green light, for example, the honey bee or flies, respectively (Backhaus, 1991; Lunau, 2014; Hannah et al., 2019); however, many beetles possess two photoreceptors, which is also likely true for Staphilinidae (beetles often found in fruit bodies), but some beetles have four photoreceptors (van der Kooi et al., 2021); birds have four kinds of photoreceptors sensitive to red, blue, and green light and some are also sensitive to UV (Hart and Hunt, 2007); the visual system of slugs is most likely not able to differentiate colors due to their reduced optical system and their mostly nocturnal activity (Dimock Jr and Parno, 1981; Nishiyama et al., 2019).
Big data integration
Macroecological community ecological approaches have proved invaluable in ecological research in testing broad species diversity and functional diversity patterns (Keith et al., 2012). Utilizing large datasets, macroecological community patterns can inform about the relative effects of different predictor sets while incorporating a large volume of existing diversity. Despite their correlative nature, the large amount of data used provides robust statistical means to gain first insights into ecological relations that are of importance to inform more detailed analyses and experiments. Existing extensive species distribution datasets that draw from reputable sources such as the CLIMFUN project (Andrew et al., 2017; Krah et al., 2019), GBIF (GBIF, 2017; Krah et al., 2023), and GlobalFungi (Větrovskỳ et al., 2020) should be integrated with reflectance measurements to build macroecological models and test for the relative importance of abiotic and biotic predictor sets (e.g., Dalrymple et al., 2020). Additionally, fungi–animal datasets in combination with reflectance data should be exploited. One example is the dataset comprising 58 fungal species, 40 insect species (Cisidae), and 65,675 interaction data points (Reibnitz, 1999), which was used prior to studying fungi–insect relationships, but without consideration of color traits (Thorn et al., 2015). In addition, several articles exist where feces were collected and fungi were identified via metagenomic approaches (Bradshaw et al., 2022; Kitabayashi et al., 2022; Ogórek et al., 2022; Weinstein et al., 2022); however, these data have not been analyzed yet in terms of the fruit body coloration. One promising approach might be to use the existing species-by-species fungus–animal associations and use occurrence data (e.g., GBIF) to test for phenological overlap of fruiting and, e.g., insect activity. Given enough temporal resolution of the data, it would be important to test for phenological mismatches over time, as this would be the first evidence for a novel form of mismatch that might be explained by climate warming.
Experiments
The attraction, aposematism, or camouflage hypothesis for fungi have not been tested in experimental settings. Therefore, real-world field experiments should be used to test the independent effects of fruit body traits on animal interactions. Furthermore, if the studied species are involved in fungus–animal interactions, color changes via abiotic factors may disrupt those interactions. One experiment could be designed to test if species with specific colors are more visited by animals. Fruit bodies could be treated in the field to disentangle a visual signal (color), an olfactory signal (volatiles), and abiotic factors by differentially excluding either the visual signal or the olfactory signal. This experiment could comprise fruit bodies of species and five treatments: (i) real fruit body, (ii) real fruit body covered by transparent odor-impermeable nylon-resin oven bag (visual only), (iii) only transparent odor-impermeable nylon-resin oven bag (visual control), (iv) real fruit body covered by odor-permeable intransparent green muslin bag (scent only), and (v) only odor-permeable intransparent green muslin bag (scent control). This approach has been successfully conducted within the plant–pollinator research (Policha et al., 2016). To identify insects and other animals, a camera survey (Schmid et al., 2019) or sticky traps (Graf et al., 2022) could be used.
Functional studies
Many of the pigments responsible for fruit body colors may have a role in resistance to abiotic stressors, including extremely high or low temperatures, low water availability, rainfall as physical damage, or ultraviolet radiation (Cordero and Casadevall, 2017). Depending on the pigment composition and the color spectra, species and individuals should be differentially able to exist in an environment more or less affected by such stressors. Thus, pigments and colors should provide a fitness advantage in harsher environments. To fully support any of the thermal-melanism, the DPH or the PPH requires three aspects to be supported (following Cordero et al., 2018): (1) colors are related to the specific abiotic variable (e.g., darker fruit bodies in colder environments), (2) colors function to reduce abiotic stress (e.g., darker fruit bodies warm faster than light-colored ones), and (3) colors provide a fitness advantage in the abiotic condition (e.g., darker fruit bodies have the same biomass in cold environments as light-colored ones in warm environments). Fruit bodies could be collected across different environments with measurements of dry weight and the number of spores determined as relative fitness. For the abiotic physiological stress experiments, fruit bodies could be subjected to high UV radiation followed by a spore viability test, or placed under an artificial solar radiation (Krah et al., 2021) to determine warming rates, or placed in a drying cabinet to determine desiccation rates.
Phylogenetics and evolution
Further phylogenetic analyses would be helpful to answer some important evolutionary questions. For example, how conserved pigments and colors are across larger phylogenies may inform about selective pressures. Furthermore, fungal diversification rates may be explained by the kind of fungus–animal interaction. Likewise, estimating precisely the first occurrence of certain pigments and colors can help to explain the mechanisms behind color pattern we see today.
Conclusion
The role of colors is a key trait with implications for fungi’s relations to both the abiotic and the biotic world. Establishing a trait-based mechanistic understanding of how color mediates fungal responses to climate and other factors will enhance our ability to forecast fungi under global change. Furthermore, identifying the role of color in fungi–animal interactions will allow assessing the potential for climate change-induced disruption of their interaction via color change. Finally, a better understanding of the biology of fruit body colors will also benefit a more holistic understanding of color across kingdoms.
Author contributions
F-SK: Conceptualization, Writing – original draft, review and editing, Funding acquisition.
Funding
The author(s) declare financial support was received for the research, authorship, and/or publication of this article. Funded by the Deutsche Forschungsgemeinschaft (DFG, German Research Foundation) – 491183248 and by the Open Access Publishing Fund of the University of Bayreuth.
Conflict of interest
The author declares that the research was conducted in the absence of any commercial or financial relationships that could be construed as a potential conflict of interest.
Publisher’s note
All claims expressed in this article are solely those of the authors and do not necessarily represent those of their affiliated organizations, or those of the publisher, the editors and the reviewers. Any product that may be evaluated in this article, or claim that may be made by its manufacturer, is not guaranteed or endorsed by the publisher.
References
Afroz Toma M., Rahman M. H., Rahman M. S., Arif M., Nazir K. H. M. N. H., Dufossé L. (2023). Fungal pigments: carotenoids, riboflavin, and polyketides with diverse applications. J. Fungi 9, 454. doi: 10.3390/jof9040454
Andrew C., Heegaard E., Kirk P. M., Bässler C., Heilmann-Clausen J., Krisai-Greilhuber I., et al. (2017). Big data integration: Pan-European fungal species observations’ assembly for addressing contemporary questions in ecology and global change biology. Fungal Biol. Rev. 31, 88–98. doi: 10.1016/j.fbr.2017.01.001
Backhaus W. (1991). Color opponent coding in the visual system of the honeybee. Vision Res. 31, 1381–1397. doi: 10.1016/0042-6989(91)90059-E
Bekiaris G., Tagkouli D., Koutrotsios G., Kalogeropoulos N., Zervakis G. I. (2020). Pleurotus mushrooms content in glucans and ergosterol assessed by ATR-FTIR spectroscopy and multivariate analysis. Foods 9, 535. doi: 10.3390/foods9040535
Borgmann-Winter B. W., Stephens R. B., Anthony M. A., Frey S. D., D’Amato A. W., Rowe R. J. (2023). Wind and small mammals are complementary fungal dispersers. Ecology 104, e4039. doi: 10.1002/ecy.4039
Bradshaw A. J., Autumn K. C., Rickart E. A., Dentinger B. T. M. (2022). On the origin of feces: Fungal diversity, distribution, and conservation implications from feces of small mammals. Environ. DNA 4, 608–626. doi: 10.1002/edn3.281
Brodie J., Ingham C. J., Vignolini S. (2021). Does structural color exist in true fungi? J. Fungi 7, 141. doi: 10.3390/jof7020141
Caro T. (2017). Wallace on coloration: contemporary perspective and unresolved insights. Trends Ecol. Evol. 32, 23–30. doi: 10.1016/j.tree.2016.10.003
Cheung P. C. (2013). Mini-review on edible mushrooms as source of dietary fiber: Preparation and health benefits. Food Sci. Hum. Wellness 2, 162–166. doi: 10.1016/j.fshw.2013.08.001
Chittka L., Menzel R. (1992). The evolutionary adaptation of flower colours and the insect pollinators’ colour vision. J. Comp. Physiol. A 171, 171–181. doi: 10.1007/BF00188925
Chittka L., Raine N. E. (2006). Recognition of flowers by pollinators. Curr. Opin. Plant Biol. 9. doi: 10.3389/fevo.2021.698137
Cordero R. J. B., Casadevall A. (2017). Functions of fungal melanin beyond virulence. Fungal Biol. Rev. 31, 99–112. doi: 10.1016/j.fbr.2016.12.003
SCordero R. J. B., Robert V., Cardinali G., Arinze E. S., Thon S. M., Casadevall A. (2018). Impact of yeast pigmentation on heat capture and latitudinal distribution. Curr. Biol. 28, 2657–2664.e3. doi: 10.1016/j.cub.2018.06.034
Cuthill I. C., Allen W. L., Arbuckle K., Caspers B., Chaplin G., Hauber M. E., et al. (2017). The biology of color. Science 357, eaan0221. doi: 10.1126/science.aan0221
Dalrymple R. L., Kemp D. J., Flores-Moreno H., Laffan S. W., White T. E., Hemmings F. A., et al. (2020). Macroecological patterns in flower colour are shaped by both biotic and abiotic factors. New Phytol. 228, 1972–1985. doi: 10.1111/nph.16737
Dawson T. P., Jackson S. T., House J. I., Prentice I. C., Mace G. M. (2011). Beyond predictions: biodiversity conservation in a changing climate. Science 332, 53–58. doi: 10.1126/science.1200303
Delhey K. (2019). A review of Gloger’s rule, an ecogeographical rule of colour: Definitions, interpretations and evidence. Biol. Rev. 94, 1294–1316. doi: 10.1111/brv.12503
Dimock R. V. Jr., Parno J. R. (1981). Bi-modal sensitivity to monochromatic light by the mud snail Ilyanassa obsoleta. Mar. Freshw. Behav. Phy 7, 291–296. doi: 10.1080/10236248109386990
Elliott T. F., Truong C., Jackson S., Zúñiga C., Trappe J., Vernes K. (2022). Mammalian mycophagy: a global review of ecosystem interactions between mammals and fungi. Fungal System. Evol. 9, 99–159. doi: 10.3114/fuse.2022.09.07
Finlay B. J., Clarke K. J. (1999). Ubiquitous dispersal of microbial species. Nature 400, 828. doi: 10.1038/23616
Floudas D., Binder M., Riley R., Barry K., Blanchette R. A., Henrissat B., et al. (2012). The paleozoic origin of enzymatic lignin decomposition reconstructed from 31 fungal genomes. Science 336, 1715–1719. doi: 10.1126/science.1221748
Fogel R., Trappe J. M. (1978). Fungus consumption (mycophagy) by small animals. Northwest Sci. 52, 1–31.
Galante T. E., Horton T. R., Swaney D. P. (2011). 95% of basidiospores fall within 1 m of the cap: a field-and modeling-based study. Mycologia 103, 1175–1183. doi: 10.3852/10-388
GBIF: The Global Biodiversity Information Facility. (2023). Available at: https://www.gbif.org/what-is-gbif.
Gill M. (1999). Pigments of fungi (Macromycetes). Natural Prod. Rep. 16, 301–317. doi: 10.1039/A705730J
Gill S. S., Tuteja N. (2010). Reactive oxygen species and antioxidant machinery in abiotic stress tolerance in crop plants. Plant Physiol. Biochem. 48, 909–930. doi: 10.1016/j.plaphy.2010.08.016
Golan J. J., Pringle A. (2017). Long-distance dispersal of fungi. Microbiol. Spectr. 5. doi: 10.1128/microbiolspec.funk-0047-2016
Graf M., Seibold S., Gossner M. M., Hagge J., Weiss I., Baessler C., et al. (2022). Coverage based diversity estimates of facultative saproxylic species highlight the importance of deadwood for biodiversity. For. Ecol. Manage. 517. doi: 10.1016/j.foreco.2022.120275
Guarro J., Gené J., Stchigel A. M. (1999). Developments in fungal taxonomy. Clin. Microbiol. Rev. 12, 454–500. doi: 10.1128/CMR.12.3.454
Guevara R., Dirzo R. (1999). Consumption of macro-fungi by invertebrates in a Mexican tropical cloud forest: do fruit body characteristics matter? J. Trop. Ecol. 15, 603–617. doi: 10.1017/S0266467499001042
Halbwachs H., Simmel J., Bässler C. (2016). Tales and mysteries of fungal fruiting: How morphological and physiological traits affect a pileate lifestyle. Fungal Biol. Rev. 30, 36–61. doi: 10.1016/j.fbr.2016.04.002
Hallenberg N., Kuffer N. (2001). Long-distance spore dispersal in wood-inhabiting basidiomycetes. Nordic J. Bot. 21, 431–436. doi: 10.1111/j.1756-1051.2001.tb00793.x
Hannah L. (2010). A global conservation system for climate-change adaptation. Conserv. Biol. 24, 70–77. doi: 10.1111/j.1523-1739.2009.01405.x
Hannah L., Dyer A. G., Garcia J. E., Dorin A., Burd M. (2019). Psychophysics of the hoverfly: categorical or continuous color discrimination? Curr. Zool. 65, 483–492. doi: 10.1093/cz/zoz008
Hannecker A., Huymann L., Hammerle F., Peintner U., Siewert B. (2023). Photochemical defense as trait of fungi from Cortinarius subgenus Dermocybe. Photochem. Photobiol. Sci. 22, 147–157. doi: 10.1007/s43630-022-00305-0
Hart N. S., Hunt D. M. (2007). Avian visual pigments: characteristics, spectral tuning, and evolution. Am. Nat. 169, S7–S26. doi: 10.1086/510141
Jacobs G. H. (2009). Evolution of colour vision in mammals. Philos. Trans. R. Soc. B: Biol. Sci. 364, 2957–2967. doi: 10.1098/rstb.2009.0039
Jaeger R. J. R., Spiteller P. (2010). Mycenaaurin A, an antibacterial polyene pigment from the fruiting bodies of Mycena aurantiomarginata. J. Natural Prod. 73, 1350–1354. doi: 10.1021/np100155z
Kalra R., Conlan X. A., Goel M. (2020). Fungi as a potential source of pigments: Harnessing filamentous fungi. Front. Chem. 8, 369. doi: 10.3389/fchem.2020.00369
Keith S. A., Webb T. J., Böhning-Gaese K., Connolly S. R., Dulvy N. K., Eigenbrod F., et al. (2012). What is macroecology? Biol. Lett. 8, 904–906. doi: 10.1098/rsbl.2012.0672
Kitabayashi K., Kitamura S., Tuno N. (2022). Fungal spore transport by omnivorous mycophagous slug in temperate forest. Ecol. Evol. 12, e8565. doi: 10.1002/ece3.8565
Kohler A., Kuo A., Nagy L. G., Morin E., Barry K. W., Buscot F., et al. (2015). Convergent losses of decay mechanisms and rapid turnover of symbiosis genes in mycorrhizal mutualists. Nat. Genet. 47, 410–415. doi: 10.1038/ng.3223
Krah F.-S., Bässler C. (2021). What can intraspecific trait variability tell us about fungal communities and adaptations? Mycol. Prog. 20, 905–910. doi: 10.1007/s11557-021-01708-6
Krah F. S., Büntgen U., Bässler C. (2023). Temperature affects the timing and duration of fungal fruiting patterns across major terrestrial biomes. Ecol. Lett 26 (9), 1572–1583. doi: 10.1111/ele.14275
Krah F.-S., Büntgen U., Schaefer H., Müller J., Andrew C., Boddy L., et al. (2019). European mushroom assemblages are darker in cold climates. Nat. Commun. 10, 2890. doi: 10.1038/s41467-019-10767-z
Krah F.-S., Hagge J., Schreiber J., Brandl R., Müller J., Bässler C. (2022). Fungal fruit body assemblages are tougher in harsh microclimates. Sci. Rep. 12, 1633. doi: 10.1038/s41598-022-05715-9
Krah F., Hess J., Hennicke F., Kar R., Bässler C. (2021). Transcriptional response of mushrooms to artificial sun exposure. Ecol. Evol. 11, 10538–10546. doi: 10.1002/ece3.7862
Læssøe T., Petersen J. H. (2019). Fungi of temperate Europe (Princeton and Oxford: Princeton University Press).
Lev-Yadun S. (2016). Defensive (anti-herbivory) Coloration in Land Plants (Cham: Springer International Publishing). doi: 10.1007/978-3-319-42096-7
Lilleskov E. A., Bruns T. D. (2005). Spore dispersal of a resupinate ectomycorrhizal fungus, Tomentella sublilacina, via soil food webs. Mycologia 97, 762–769. doi: 10.3852/mycologia.97.4.762
Liu S., García-Palacios P., Tedersoo L., Guirado E., van der Heijden M. G., Wagg C., et al. (2022). Phylotype diversity within soil fungal functional groups drives ecosystem stability. Nat. Ecol. Evol. 6, 900–909. doi: 10.1038/s41559-022-01756-5
Lodge D. J., Padamsee M., Matheny P. B., Aime M. C., Cantrell S. A., Boertmann D., et al. (2014). Molecular phylogeny, morphology, pigment chemistry and ecology in Hygrophoraceae (Agaricales). Fungal Diversity 64, 1–99. doi: 10.1007/s13225-013-0259-0
Lomáscolo S. B., Levey D. J., Kimball R. T., Bolker B. M., Alborn H. T. (2010). Dispersers shape fruit diversity in Ficus (Moraceae). Proc. Natl. Acad. Sci. 107, 14668–14672. doi: 10.1073/pnas.1008773107
Lunau K. (2014). Visual ecology of flies with particular reference to colour vision and colour preferences. J. Comp. Physiol. A 200, 497–512. doi: 10.1007/s00359-014-0895-1
Lunde L. F., Birkemoe T., Kauserud H., Boddy L., Jacobsen R. M., Morgado L., et al. (2022). DNA metabarcoding reveals host-specific communities of arthropods residing in fungal fruit bodies. Proc. R. Soc. B 289, 20212622. doi: 10.1098/rspb.2021.2622
McGill B. J., Enquist B. J., Weiher E., Westoby M. (2006). Rebuilding community ecology from functional traits. Trends Ecol. Evol. 21, 178–185. doi: 10.1016/j.tree.2006.02.002
Nagy L. G., Toth R., Kiss E., Slot J., Gacser A., Kovacs G. M. (2017). Six key traits of fungi: their evolutionary origins and genetic bases. Microbiol. Spectr. 5. doi: 10.1128/9781555819583.ch2
Narsing Rao M. P., Xiao M., Li W.-J. (2017). Fungal and bacterial pigments: secondary metabolites with wide applications. Front. Microbiol. 8. doi: 10.3389/fmicb.2017.01113
Nishiyama H., Nagata A., Matsuo Y., Matsuo R. (2019). Light avoidance by a non-ocular photosensing system in the terrestrial slug Limax valentianus. J. Exp. Biol. 222, jeb208595. doi: 10.1242/jeb.208595
Niskanen T., Lücking R., Dahlberg A., Gaya E., Suz L. M., Mikryukov V., et al. (2023). Pushing the frontiers of biodiversity research: unveiling the global diversity, distribution, and conservation of fungi. Annu. Rev. Environ. Resour. 48, annurev–environ-112621-090937. doi: 10.1146/annurev-environ-112621-090937
Oechler H., Krah F.-S. (2022). Response of fruit body assemblage color lightness to macroclimate and vegetation cover. Front. Ecol. Evol. 10. doi: 10.3389/fevo.2022.829981
Ogórek R., Suchodolski J., Dudek B. (2022). Droppings of Svalbard reindeer (Rangifer tarandus platyrhynchus) as a reservoir of cultivable micromycetes on Spitsbergen. Polish Polar Res. 43 (3), 247–265. doi: 10.24425/ppr.2022.140367
Policha T., Davis A., Barnadas M., Dentinger B. T., Raguso R. A., Roy B. A. (2016). Disentangling visual and olfactory signals in mushroom-mimicking Dracula orchids using realistic three-dimensional printed flowers. New Phytol. 210, 1058–1071. doi: 10.1111/nph.13855
Reibnitz J. V. (1999). Verbreitung und Lebensräume der Baumschwammfresser Südwestdeutschlands (Coleoptera: Cisidae). Mitt. Entomologischer Verein Stuttgart 34, 1–76.
Reinhold L. (2020). Fluorescent forests: of mushrooms and marsupials. Queensland Mycologist 15, 5–12.
Rensch B. (1929). Das Prinzip geographischer Rassenkreise und das Problem der Artbildung. Nature 124, 753–754. doi: 10.1038/124753a0
Sánchez-García M., Ryberg M., Khan F. K., Varga T., Nagy L. G., Hibbett D. S. (2020). Fruiting body form, not nutritional mode, is the major driver of diversification in mushroom-forming fungi. Proc. Natl. Acad. Sci. 117, 32528–32534. doi: 10.1073/pnas.1922539117
Schmid L., Bässler C., Schaefer H., Krah F.-S. (2019). A test of camera surveys to study fungus-animal interactions. Mycoscience 60, 287–292. doi: 10.1016/j.myc.2019.04.002
Seibold S., Mueller J., Baldrian P., Cadotte M. W., Stursova M., Biedermann P. H. W., et al. (2019). Fungi associated with beetles dispersing from dead wood - Let’s take the beetle bus! Fungal Ecol. 39, 100–108. doi: 10.1016/j.funeco.2018.11.016
Sherratt T. N., Wilkinson D. M., Bain R. S. (2005). Explaining Dioscorides’ “double difference’’: why are some mushrooms poisonous, and do they signal their unprofitability? Am. Nat. 166, 767–775. doi: 10.1086/497399
Siewert B. (2021). Does the chemistry of fungal pigments demand the existence of photoactivated defense strategies in basidiomycetes? Photochem. Photobiol. Sci. 20, 475–488. doi: 10.1007/s43630-021-00034-w
Sinnott-Armstrong M. A., Donoghue M. J., Jetz W. (2021). Dispersers and environment drive global variation in fruit colour syndromes. Ecol. Lett. 24, 1387–1399. doi: 10.1111/ele.13753
Spiteller P. (2015). Chemical ecology of fungi. Natural Prod. Rep. 32, 971–993. doi: 10.1039/c4np00166d
Stevens M., Merilaita S. (2009). Animal camouflage: current issues and new perspectives. Phil. Trans. R. Soc B 364, 423–427. doi: 10.1098/rstb.2008.0217
Thorn S., Mueller J., Baessler C., Gminder A., Brandl R., Heibl C. (2015). Host abundance, durability, basidiome form and phylogenetic isolation determine fungivore species richness. Biol. J. Linn. Soc. 114, 699–708. doi: 10.1111/bij.12447
Tremble K., Hoffman J. I., Dentinger B. T. M. (2023). Contrasting continental patterns of adaptive population divergence in the holarctic ectomycorrhizal fungus Boletus edulis. New Phytol. 237, 295–309. doi: 10.1111/nph.18521
Trullas S. C., van Wyk J. H., Spotila J. R. (2007). Thermal melanism in ectotherms. J. Thermal Biol. 32, 235–245. doi: 10.1016/j.jtherbio.2007.01.013
Urban M. C., Bocedi G., Hendry A. P., Mihoub J.-B., Pe’er G., Singer A., et al. (2016). Improving the forecast for biodiversity under climate change. Science 353, aad8466-1-aad8466-9. doi: 10.1126/science.aad8466
van der Kooi C. J., Stavenga D. G., Arikawa K., Belušič G., Kelber A. (2021). Evolution of insect color vision: from spectral sensitivity to visual ecology. Annu. Rev. Entomol. 66, 435–461. doi: 10.1146/annurev-ento-061720-071644
van der Niet T., Peakall R., Johnson S. (2014). Pollinator-driven ecological speciation in plants: New evidence and future perspectives. Ann. Bot. 113, 199–212. doi: 10.1093/aob/mct290
Vavricka C. J., Christensen B. M., Li J. (2010). Melanization in living organisms: a perspective of species evolution. Protein Cell 1, 830–841. doi: 10.1007/s13238-010-0109-8
Velíšek J., Cejpek K. (2011). Pigments of higher fungi-a review. Czech J. Food Sci. 29, 87–102. doi: 10.17221/524/2010-CJFS
Větrovskỳ T., Morais D., Kohout P., Lepinay C., Algora C., Awokunle Hollá S., et al. (2020). GlobalFungi, a global database of fungal occurrences from high-throughput-sequencing metabarcoding studies. Sci. Data 7, 1–14. doi: 10.1038/s41597-020-0567-7
Watson R., Baste I., Larigauderie A., Leadley P., Pascual U., Baptiste B., et al. (2019). Summary for policymakers of the global assessment report on biodiversity and ecosystem services of the Intergovernmental Science-Policy Platform on Biodiversity and Ecosystem Services (Bonn, Germany: IPBES Secretariat), 22–47.
Keywords: fungi–animal interactions, climate change, attraction, aposematism, camouflage, thermoregulation, defense, reflectance
Citation: Krah F-S (2023) The unresolved ecological and evolutionary role of fungal fruit body coloration. Front. Ecol. Evol. 11:1326710. doi: 10.3389/fevo.2023.1326710
Received: 23 October 2023; Accepted: 22 November 2023;
Published: 08 December 2023.
Edited by:
Tom N. Sherratt, Carleton University, CanadaReviewed by:
Simcha Lev-Yadun, University of Haifa, IsraelDavid Wilkinson, University of Lincoln, United Kingdom
Copyright © 2023 Krah. This is an open-access article distributed under the terms of the Creative Commons Attribution License (CC BY). The use, distribution or reproduction in other forums is permitted, provided the original author(s) and the copyright owner(s) are credited and that the original publication in this journal is cited, in accordance with accepted academic practice. No use, distribution or reproduction is permitted which does not comply with these terms.
*Correspondence: Franz-Sebastian Krah, krah@uni-bayreuth.de
†ORCID: Franz-Sebastian Krah, orcid.org/0000-0001-7866-7508