- 1Laboratory of Growth Regulators, Faculty of Science of Palacký University and Institute of Experimental Botany of the Czech Academy of Sciences, Olomouc, Czechia
- 2Department of Chemical Biology, Faculty of Science, Palacký University, Olomouc, Czechia
To cope with biotic and abiotic stress conditions, land plants have evolved several levels of protection, including delicate defense mechanisms to respond to changes in the environment. The benefits of inducible defense responses can be further augmented by defense priming, which allows plants to respond to a mild stimulus faster and more robustly than plants in the naïve (non-primed) state. Priming provides a low-cost protection of agriculturally important plants in a relatively safe and effective manner. Many different organic and inorganic compounds have been successfully tested to induce resistance in plants. Among the plethora of commonly used physicochemical techniques, priming by plant growth regulators (phytohormones and their derivatives) appears to be a viable approach with a wide range of applications. While several classes of plant hormones have been exploited in agriculture with promising results, much less attention has been paid to cytokinin, a major plant hormone involved in many biological processes including the regulation of photosynthesis. Cytokinins have been long known to be involved in the regulation of chlorophyll metabolism, among other functions, and are responsible for delaying the onset of senescence. A comprehensive overview of the possible mechanisms of the cytokinin-primed defense or stress-related responses, especially those related to photosynthesis, should provide better insight into some of the less understood aspects of this important group of plant growth regulators.
Introduction
The crisis of feeding the world’s rapidly growing population with annual growth of around 80 million (Roser et al., 2013) is compounded by a plethora of conflicting issues, such as limited water availability or insufficient allocation of agricultural land. Moreover, various biotic and abiotic stresses resulting from long-term exposure to high temperatures and osmotic stress significantly limit crop yields. Water stress and salinity are considered to be the biggest challenges of present-day agriculture (Abhinandan et al., 2018), leading to poor seed germination rates, poor seedling emergence and poor stand establishment, thereby significantly limiting global crop production (Reed et al., 2022). Sustainable agriculture under the pressure of climatic change is one of the priorities of developed countries across the globe. The crop productivity is expected to have increased by approximately 60-100% by 2050; achieving such a goal without damaging the agricultural soil is challenging (Lau et al., 2022). Environmental stresses may be prevented by optimizing plant growth conditions and applying plant growth regulators (Yakhin et al., 2016). Seed priming – a process that involves seed imbibition with small amount of water to allow distribution of the priming agent – is recognized as an innovative and affordable technology to counteract harmful effects of abiotic stress by enhancing plant defense responses (Arun et al., 2022).
Similar to immunization in animals and humans, where an infectious agent or vaccine helps the immune system develop immunity to a disease, plants can also be immunized. This type of “acquired physiological immunity” in plants is now referred to as priming and can generally be described as the ability to elicit a faster and/or stronger defense response after a prior exposure to a biotic or an abiotic stress condition (Bruce et al., 2007; Hilker and Schmülling, 2019). Both biotic and abiotic stressors can elicit an adaptive response of the plant immune system, including changes at the epigenetic level, transcriptional reprogramming, and changes in protein phosphorylation (Conrath, 2011; Mauch-Mani et al., 2017). In general, priming allows plants to remember past stress events and prepare them for future attacks (Tsuda et al., 2013; Bjornson et al., 2014; Choi et al., 2014; Campos et al., 2016; de Zelicourt et al., 2016; Ariga et al., 2017; Chen et al., 2021). Linked multilevel processes generate a stress imprint or stress memory of a given priming event so that when a plant encounters the same specific condition, it can reach a primed state of enhanced and faster responsiveness and effectively regulate the relevant defense signaling cascade(s) (Conrath et al., 2006). Some excellent reviews address the molecular mechanisms underlying stress memory and priming formation upon various stress conditions in different plant species (Conrath, 2011; Conrath et al., 2015).
While defense priming is typically associated with bacterial and fungal pathogens or other biotic stressors, an altered response to abiotic stressors (also called hardening) is no less important. Previous exposure to a mild abiotic stress including heat, cold, osmotic or water stress can also improve resistance of various environmental stresses (Thomashow, 2010; Amooaghaie and Tabatabaie, 2017; Fan et al., 2018; Lemmens et al., 2019). Priming with plant hormones (hormopriming) is another popular and effective approach how to improve overall plant development (Iqbal et al., 2006; Nawaz et al., 2013; Chipilski et al., 2021; Vedenicheva et al., 2022), with cytokinins (CKs) emerging as a potentially interesting new group of hormopriming regulators that have been repeatedly shown to influence several developmental processes, including photosynthesis and senescence (Sobol et al., 2014; Vylíčilová et al., 2016; Hönig et al., 2018; Kučerová et al., 2020). In this review, we focus on possible CK effects in stress priming, with particular emphasis on the maintenance of photosynthesis, which is likely the backbone of the adaptive response mediated by this plant hormone.
Cytokinin priming to increase crops growth and yield
It has been recently reported that priming of wheat seeds with benzylaminopurine (BAP) and kinetin (KIN) applied by spraying the wheat plants during the grain filling stage resulted in up to 14% higher productivity, higher fresh and dry weight, and chlorophyll content index of flag leaves (Chipilski et al., 2021). In addition to the positive impact on photosynthesis, another way in which CKs can influence plant fitness and yield is their natural ability to suppress oxidative stress, which has been also repeatedly associated with defense priming in plants (Kerchev et al., 2020). The offspring of CK-treated plants during the grain filling stage showed a lower accumulation of stress markers in field conditions demonstrating the transgenerational effect of this stress imprint (Chipilski et al., 2021). The CK-primed enhanced protection against oxidative damage was demonstrated on 15-day-old seedlings of wheat (cv. Geya-1) and these results positively correlated with a 25% lower average accumulation of malondialdehyde and hydrogen peroxide in 5-day-old seedlings of such wheat. In addition, the exogenous CK application in field conditions enhances the wheat seed viability after a low-temperature storage, which is an essential feature for practical agriculture (Chipilski et al., 2021). Moreover, KIN improved tomato shoot lengths after seed priming (Nawaz et al., 2013). As outlined above, the CK treatment can reasonably influence seed germination. This may be correlated with increased activity of hydrolytic enzymes in developing seeds, which can neutralize the impact of seed aging (Nawaz et al., 2013).
CK priming becomes particularly important when dealing with the consequences of climate change, such as temperature changes, increased solar radiation, drought, or salinity. The priming of rye seeds (cv. Boguslavka) with zeatin resulted in significant changes of endogenous CK pools in both shoots and roots of 7-day-old seedlings (Vedenicheva et al., 2022). Also, rye plants grown from CK-primed seeds were more resistant to hyperthermia stress than untreated control (Vedenicheva et al., 2022). Two cultivars of hexaploid spring wheat (Triticum aestivum L.) seeds were pre-soaked in BAP and KIN solutions, and the primed and non-primed seeds of salt-intolerant MH-97, as well as salt-tolerant Inqlab-91, were compared. KIN was effective in increasing the germination rate in the salt-intolerant cultivar and early seedling growth when compared with hydropriming under salt stress. Furthermore, the KIN-primed seeds showed a consistent promoting effect in the field and improved growth and grain yield in both cultivars under salt stress (Iqbal et al., 2006). The authors explain the priming effect by a complex crosstalk regulatory mechanism involving levels of active CK, abscisic acid (ABA) and indoleacetic acid (IAA) in developing plant leaves. CKs applied on the seeds can enhance the future shoot regeneration efficiency, as it activates the dedication of the shoot progenitor at later stages and allows chromatin to maintain shoot identity genes (Fathy et al., 2022; Wu et al., 2022).
Many CK-based compounds have already found application in micropropagation techniques and protection of plants against various types of abiotic stress, some were also tested experimentally in in field trials (Koprna et al., 2016; Plíhalová et al., 2016). Regarding seed priming, it may be interesting to note that the application of some CK-based derivatives, such as 2-substituted-6-anilino-9-heterocyclylpurine derivatives, has already been patented for seed dressing/coating of various crops, mainly cereals (Zatloukal et al., 2015). Their application led to increase of yield and quality of the agricultural product in harmful conditions (Zatloukal et al., 2015). Purine based (CK) derivatives were applied on maize, winter wheat and rapeseed. Other CK derivatives derived from urea were applied to fight stress caused by drought, heat or cold stress and salinity (Nisler et al., 2015). Such urea-based derivatives were applicated on winter wheat Triticum aestivum cv Hereward and spring barley (Hordeum vulgare), malting variety Bojos or winter oilseed rape treated by picking with a 50 µM solution of urea-based CK derivatives (Nisler et al., 2015). Since even seed coating where the active substances remain on the surface of the seed can cause such an impact on the crop productivity as described above, soaking the seed in the priming agents (although more technically demanding) may produce earlier and more consistent responses. Thus, it can have a more significant impact on crop yield.
Mechanisms of cytokinin-mediated effects on photosynthesis
The reduction in photosynthetic capacity is associated with the reduced growth in many plant species exposed to stressful environments, demonstrating a direct relation between the photosynthetic capacity and crop yield (Moradi and Ismail, 2007; Naeem et al., 2010; Wu et al., 2012; Wu et al., 2019). Among the various biochemical processes, photosynthesis is highly sensitive to any environmental stress, with the photosynthetic apparatus being one of the most stress-sensitive plant components. Thus, photosynthetic capacity determined through the gaseous exchange (von Caemmerer and Farquhar, 1981) and chlorophyll fluorescence (Roháček, 2002; Lazár, 2015) measurements provides an excellent way to analyze the effects of stress on plants. In addition, monitoring photosynthetic variables by non-invasive techniques can be used to assess whether priming effects have been achieved and to determine the potential fitness benefits of the process. Optimization of the photosynthesis through transcriptomic reprogramming of target components of photosynthetic protein complexes or specific regulation of chlorophyll-related and other metabolic processes could, on the other hand, provide us with a powerful tool to increase yield under certain stress conditions, resulting in an overall improvement of agricultural production.
Priming, due to an inherently relatively high stability of photosystems and their high recovery response, offers a role in reducing the severity of stress and can therefore promote a rapid and complete recovery of plant physiological functions. Although different priming methods undoubtedly improve photosynthetic parameters and overall photosynthetic efficiency of plants exposed to various environmental stresses (Vincent et al., 2020; Sorrentino et al., 2021; Aswathi et al., 2022; Johnson and Puthur, 2022), the control mechanisms that govern the photosynthetic protection are still poorly understood. Recently, a major role in both the direct and indirect regulation of the photosynthetic protection under stress conditions has been attributed to various plant hormones (Müller and Munné-Bosch, 2021), which have long been known as effective priming agents (Kauss and Jeblick, 1995; Mur et al., 1996; Ton and Mauch-Mani, 2004; Hirao et al., 2012; Wang et al., 2014; Ji et al., 2021). We observed that CKs and their derivatives have important protective effects on the photosynthetic apparatus, which is manifested by the upregulation of photosystem components PSII (and more rarely PSI), upregulation of Calvin cycle components (RuBisCO, glyceraldehyde-3-phosphate dehydrogenase, etc.) and maintenance of chlorophyll through the downregulation of chlorophyll catabolism (chlorophyll b reductase coded by NOL, NYE1, NYE2, etc.; Vylíčilová et al., 2016) and the upregulation of chlorophyll biosynthesis, presumably through upregulation of protochlorophyllide oxidoreductase (Kusnetsov et al., 1998). This leads to a significantly better plant fitness and a delayed onset of senescence.
CKs are non-volatile plant hormones that reside mostly within plant vascular tissues and are unable to provide defense responses in neighboring plants (Dervinis et al., 2010). However, this does not seem to lessen their potential as effective priming agents. Apart from the relatively decent knowledge about their mode of action under optimal developmental and growth conditions, their role in plant defense priming related especially to photosynthesis is still a mosaic of individual findings. CKs play a central role in the chloroplast development and function and in chlorophyll biosynthesis (Cortleven and Schmülling, 2015). They are known to regulate many genes associated with photosynthesis (Brenner and Schmülling, 2012) and protect the photosynthetic machinery and productivity of plants exposed to various stresses (Chernyad’ev, 2009). CKs appear to act in the protection of photosynthesis at both levels, light and dark photosynthetic reactions, including the control of gas exchange.
Regarding the control of gas exchange by CKs, they are often considered as an ABA antagonist. Generally, exogenous CKs can inhibit ABA-induced stomatal closure in diverse species (Tanaka et al., 2006). The increased CK concentration in the xylem sap promotes the opening of stomata and reduces sensitivity to ABA (Daszkowska-Golec and Szarejko, 2013). Wild-type tomato leaves treated with CK showed enhanced transpiration and increased numbers of stomata per leaf area than untreated leaves (Farber et al., 2016). Mohammadi et al. (2015) demonstrated that BAP foliar application can significantly increase stomatal conductance in wheat under drought stress. An increase in internal CO2 concentration and water use efficiency (WUE) or increase in CO2 assimilation rate, stomatal conductance and transpiration was recorded under salt stress in eggplant after seedlings’ exposition to BAP (Wu et al., 2012) or in Panax ginseng plants at a later growth stage after inserting the stem base into BAP solution (Li and Xu, 2014). The naturally occurring zeatin-type bases, ribosides and O-glucosides supplied to the leaf in xylem sap regulated transpiration in planta in oat (Badenoch-Jones et al., 1996). Wheat priming by seed pretreatment with cis-zeatin or trans-zeatin significantly increased stomatal conductance, photosynthetic efficiency, shoot biomass with grain yield upon salt and drought stress (Alharby et al., 2020). Applying synthetic cytokinin, KIN, to Tradescantia albiflora leaves induced stomatal opening (Pharmawati et al., 1998). Seed priming with KIN alleviated the adverse effect of salt stress on gas exchange characteristics of wheat leading to improving growth and grain yield (Iqbal and Ashraf, 2005). Similarly, foliar spray of KIN on salinized mulberry plants increased the net photosynthetic rate (Pn), WUE, carboxylation efficiency and leaf yield (Das et al., 2002). KIN could act as an effective priming agent also upon waterlogging stress, as reduced levels of reactive oxygen species (ROS), better water status, osmotic adjustment and an increased Pn, WUE, improved growth and biomass under waterlogging were detected in primed mungbean plants (Islam et al., 2022a). Foliar-applied KIN remarkably improved maize performance by modulating growth, gas exchange and water related parameters under drought stress (Islam et al., 2022b).
The mechanism of direct action of CK on guard cells may involve the induction of membrane hyperpolarization by stimulation of the electrogenic H+-pump (Pospíšilová, 2003). The internal cytosolic free calcium concentration may mediate interactions between CK and ABA (Hare et al., 1997). In Kentucky Bluegrass, BAP has been proposed to promote stomatal opening through its effect on ABA balance leading to improved photosynthetic recovery from drought (Hu et al., 2012). CKs may promote stomatal opening also by scavenging H2O2 in guard cells as demonstrated in Vicia faba plants (Song et al., 2006). In addition, a higher carboxylation efficiency (Pn/Ci) was observed in Anthurium plants sprayed with KIN along with its possible effects on gas exchange and antioxidant enzyme activities (de Moura et al., 2018). Farber et al. (2016) proposed that CKs can act indirectly in stomata movement – CK levels reduced during adaptation to water deficiency suppress growth and reduce stomatal density, both of which reduce transpiration, thereby increasing the tolerance to drought. Priming by exogenous application of BAP to nutrient solution upregulated the RuBisCO large subunit content in some leaves of wheat plants (Criado et al., 2009). An incubation of wheat leaves in BAP solution reduced the degradation of the large and small subunits of RuBisCO (Zavaleta-Mancera et al., 2007). Foliar spraying of rice by synthetic CK (N-2-(chloro-4-pyridyl)-N-phenyl urea) reversed the drought mediated suppression of RAF1 and the RuBisCO activase proteins, implied in the assembly and activation of RuBisCO complex (Gujjar et al., 2020). Thus, CKs may also enhance photosynthesis at the molecular level by modulating the abundance of proteins related to stomatal conductance, chlorophyll content, and RuBisCO activity (Gujjar et al., 2020).
CKs may also protect the primary photochemistry processes of photosynthesis. An improved photosynthetic performance at donor and acceptor sides of the photosystem II reaction centre (RCII) was detected in wheat plants sprayed with BAP, which simultaneously increased their endogenous zeatin levels. A higher effective and maximal quantum yields of PSII photochemistry in the light (ΦPSII) and dark (Fv/Fm) adapted state, better transfers of electrons beyond QA, enhanced electron transport rate and lower relative variable fluorescence intensity at the J-step were detected in the BAP primed wheat (Yang et al., 2018). In drought stressed maize seedlings, exogenous BAP priming regulated transient rise of fluorescence and increased the electron donation capacity of PSII (Shao et al., 2010). Application of BAP to the nutrient solution alleviated the detrimental effect of salt stress on primary photochemistry of eggplant by increasing Fv/Fm, Fv´/Fm´ (maximal quantum yield of PSII photochemistry in light adapted state), ΦPSII and increase of qp (parameter of photochemical quenching) at the expense of non-photochemical quenching (NPQ) leading to a lower dissipation of excitation energy in the PSII antennae (Wu et al., 2012).
The protection of primary photochemistry by CKs can be attributed to their positive effect on the maintenance of chlorophyll pigments and the integrity of chloroplast membranes. Priming with BAP prior to water stress resulted in increased content of xanthophyll cycle pigments and their degree of de-epoxidation in four drought-exposed plant species (Haisel et al., 2006). Exogenously applied BAP significantly reduced the senescence-induced decrease in Chl, car, xanthophyll content and in the Chl/car ratio which was reflected in a lower impairment of PSII function in barley segments (Janečková et al., 2019). Seed priming by BAP significantly increased the content of photosynthetic pigments (chl a+b), car and carbohydrates in a salt stressed soybean (Mangena, 2020). Other mechanisms by which CKs maintain PSII functionality could be to stabilize Chl-protein complexes, both Light-harvesting complex II (LHCII) and RCII, as demonstrated during dark-induced senescing Arabidopsis leaves floating in BAP solution (Oh et al., 2005). In line with this, several genes encoding the light-harvesting chlorophyll a/b-binding proteins and various proteins of PSII are regulated by CKs in Arabidopsis (Brenner and Schmülling, 2012; Vylíčilová et al., 2016). CKs may also protect the cell membranes and the photosynthetic machinery from oxidative damage. Exogenous BAP reduced levels of ROS and enhanced the activity of antioxidant enzymes (CAT, APX) in dark senescing wheat leaves (Zavaleta-Mancera et al., 2007). Exogenously applied BAP alleviated the harmful effects of salt stress by increasing photosynthetic efficiency and activity of antioxidant enzymes (SOD, POD, APX, CAT) and by reducing malonaldehyde contents and O2.- production in eggplant (Wu et al., 2012). Foliar application of KIN to Vigna radiata plants regulated antioxidant enzyme activities and reduced the increase in total peroxide, leading to suppression of the adverse effect of salt stress (Chakrabarti and Mukherji, 2003).
In addition to naturally occurring CKs or the notorious synthetic derivatives, a new avenue opens up for the possibility of studying the priming of the photosynthetic apparatus using purposefully modified CK derivatives. Exogenous KIN and its synthetic derivatives significantly protected lipid membranes from the negative effects of accumulated ROS in detached wheat leaves in the dark (Mik et al., 2011). Synthetic purine based halogenated CK derivatives increased PSII photochemistry efficiency, chlorophyll and carotenoid levels, and abundance of some LHCII components during Arabidopsis leaf senescence (Vylíčilová et al., 2016). Aromatic CK arabinosides (BAPAs) presumably act as a new type of priming agents that promote the plant innate immunity (PAMP-like responses) and positively affect leaf longevity (Bryksová et al., 2020). BAPAs treatment in detached Arabidopsis leaves indicated its action as a priming agent for the PTI (PAMP-triggered immunity) response (Bryksová et al., 2020) and at the same time markedly reduced the high light-induced cell membrane damage, accumulation of lipid hydroperoxides as well as PSII photoinhibition (Kučerová et al., 2020).
Cytokinin crosstalk with other phytohormones in priming
At first glance, the role of CKs in plant defense and priming may seem auxiliary compared to other plant hormones and volatile compounds. The traditional plant defense response related to the hormones salicylic acid (SA), jasmonic acid (JA) and ethylene (ET) are interlinked in a complex network regulating plant immunity (Conrath, 2006; Mishina and Zeier, 2007; Pieterse et al., 2009; Engelberth et al., 2011; Fragnière et al., 2011; Pieterse et al., 2014). These compounds and their volatile derivatives are well-documented as priming agents in many physiological processes (Farmer and Ryan, 1990; Engelberth et al., 2011; Song and Ryu, 2018; Avramova, 2019; Zhao et al., 2020; Singewar et al., 2021). In last decades, the participation of CKs in biotic (Choi et al., 2011; Cortleven et al., 2019) and abiotic (Barciszewski et al., 2000; Wani et al., 2016) stress responses has also been demonstrated; however, less is known about the molecular aspects of CKs in priming. Similarities in the defense mechanisms mediated by CKs and other hormones or Green Leaf Volatiles (GLVs) suggest that CKs play a more important role in the priming process than previously thought.
CKs are essential in orchestrating an immune response, most likely through the crosstalk mechanisms with other hormonal pathways. Importantly, CKs are significantly involved in plant-pathogen interactions through crosstalk with the SA pathway (reviewed in Choi et al., 2011). The CK transcription factor ARR2 confers resistance to Pseudomonas syringae in Arabidopsis via interaction with SA response factor TGA3 and binding to the PR1 promoter. In addition to the direct activation of defense responses by ARR2, CK pretreatment led to a hyperactivation of PR1 transcription after pathogen inoculation (Choi et al., 2010). It was also shown that high concentrations of CK primed defense responses in Arabidopsis seedlings against the Hyaloperonospora arabidopsidis (Argueso et al., 2012). CK treatment alone resulted in slightly increased SA-related defense pathway gene expression. However, following the pathogen inoculation, it was further potentiated. The CK-responsive gene expression was diminished in eds16 mutant plants with impaired SA biosynthesis. Thus, this crosstalk mechanism may help plants fine-tune the defense responses against biotrophic pathogens and adjust the effects of CK on plant immunity (Argueso et al., 2012).
In addition to the well-documented crosstalk with the SA pathway, the connection of the CK pathway with the JA pathway has also been reported. CKs can prime an immune response against herbivory through a crosstalk with the JA pathway, resulting in reduced emission of GLVs (Schäfer et al., 2015). GLVs were among the first well-documented compounds to effectively prime a plant’s defense system (Engelberth et al., 2004). In planta exposure to GLV cis-3-hexenyl acetate caused a rapid accumulation of JA and linolenic acid (LNA) in poplar leaves after herbivory (Frost et al., 2008). In line with this, Dervinis et al. (2010) reported higher JA and LNA levels after the CK pretreatment following wounding, while the CK treatment alone did not alter the JA and LNA levels in unwounded leaves. In addition, wounding and CK priming resulted in reduced larval weight gain (Dervinis et al., 2010).
Elevated levels of JA were also measured in a recent study on a CK-mediated resistance against brown planthopper in rice (Zhang et al., 2022). Using the CRISPR-Cas9 genome-editing approach, the authors generated two independent rice knock-out lines of cytokinin oxidase/dehydrogenase 1 (OsCKX1). Consequently, the mortality dropped to 30% in the case of OsCKX1 knock-out plants upon an infestation by brown planthopper, compared to the 90% wild-type mortality upon infestation. A simple CK treatment positively regulated JA biosynthetic and an expression of JA-responsive genes. In addition, the CK-mediated resistance against the brown planthopper infestation was diminished in JA-deficient mutant og1 (Zhang et al., 2022). Although previous studies have shown an antagonistic relationship between these two hormones, in the case of biotic stress defense responses and priming, the roles of CK and JA may be rather synergistic (Zhang et al., 2022).
The mechanisms of a crosstalk between GLV and CK pathways are much less understood but offer a significant potential for further study. It is tempting to speculate that there should be a regulatory mechanism to maintain a balance in leaf mining processes during herbivory. The existence of “green islands” with elevated levels of zeatins, isopentenyl adenine and other CKs has been reported during leaf-mining by galling insects (Giron et al., 2007). Colonization of plants by endophagous organisms thus requires a hormonal crosstalk, with GLVs playing a possible role in the process due to their volatile nature, which allows them to prime defense responses in places where CKs cannot overcome the natural barriers given by vascular constraints. Interestingly, after the treatment of Nicotiana attenuata leaves with the oral secretion of Manduca sexta, the CK pathway suppressed GLV esters emission (Schäfer et al., 2015). While JA concentrations are positively correlated with the activity of the CK pathway (Dervinis et al., 2010; Schäfer et al., 2015), the release of GLV esters was negatively correlated, suggesting that CKs control the balance between these two oxylipin classes (Schäfer et al., 2015).
As outlined by Schäfer et al. (2015), the activation of the classic CK signaling pathway after a CK application does not exclude the possibility of an alternative CK pathway involved in plant defense and priming. Priming enables plants to retain a “stress memory” and the priming state can last for multiple generations (Holeski et al., 2012; Hilker and Schmülling, 2019). For this reason, CK priming may also trigger various epigenetic modifications. CK-induced phenotypic changes in canola, such as increased surface area of petals, jagged edges of petals, and altered vasculature of flowers, are carried forward to the next generation of non-primed plants (Zuñiga-Mayo et al., 2018). This phenomenon can be achieved through the alterations of DNA methylation status. S-Adenosyl-L-homocysteine hydrolase (SAHH) appears to play a major role in this process (Li et al., 2008). Exogenous CK application induced the expression of the three cytosine DNA methyltransferase genes, MET1, CMT3, and DRM2, suggesting an important role of CKs in promoting DNA methylations in Arabidopsis (Li et al., 2008). Interestingly, downregulation of SAHH caused DNA hypomethylation, increased levels of CKs, and resistance against various plant viruses in tobacco (Masuta et al., 1995).
In addition, our aromatic CK derivatives that have been synthesized and tested retain certain properties of CK responses, such as delayed senescence and defense against pathogens, while the classical CK signaling pathway is only negligibly affected (Bryksová et al., 2020; Kučerová et al., 2020); therefore, an alternative mode of action for CK priming via the classical MAPK signaling cascade in PAMP-triggered immunity (PTI) responses and underlying epigenetic modifications cannot be ruled out. Experiments with these aromatic CK derivatives confirmed the synergistic relationship between CK and JA in defense priming, as the levels of JA and its metabolites were significantly elevated (Bryksová et al., 2020). However, a negative correlation was found between the senescence activity of aromatic CK derivatives and ET production (Kučerová et al., 2020). An ET production is generally known to be stimulated by exogenous CK treatment (Cary et al., 1995; Zdarska et al., 2015). Together with the JA pathway, ET regulates the herbivory-induced responses (Onkokesung et al., 2010). Interestingly, no stimulation of ET production was observed in aromatic CK derivative-treated wheat and Arabidopsis leaves (Kučerová et al., 2020).
While the effects of CK priming under biotic stresses are relatively well documented, mechanistic studies on CK priming in relation to abiotic stresses are just beginning to emerge. Previous works are mainly devoted to CK’s role in priming against heat stress and drought (Cheikh and Jones, 1994; Kang et al., 2012; Sobol et al., 2014; Wu et al., 2016; Wu et al., 2017). Kang et al. (2012) found that sensor histidine kinase (AHK) mutant lines, mainly ahk2 and ahk3, show significant tolerance to dehydration. CK crosstalk with ABA may be significantly involved in dehydration tolerance, as ahk mutants have an increased level of expression of ABA-upregulated genes (Kang et al., 2012). CK pretreatment with KIN was able to further increase the survival rate of ahk single mutant lines after subsequent dehydration stress (Kang et al., 2012). This phenomenon can be explained by the interplay of increased expression of ABA-upregulated genes and a wide range of CK effects (including priming) in plant defense may not be linked to the classical CK pathway, as previously discussed.
Conclusion and perspectives
In the last decade, many studies have pointed to the practical use of plant growth regulators that can mitigate the negative effects of biotic and abiotic stress conditions and improve overall crop condition and yield. Hormopriming is widely accepted as an efficient way to create memory imprints in seeds or young developing plants that can later, under limiting or stressful conditions, help shift the endogenous hormonal balance towards defense responses. Given the persistent social barriers to genetically modified crops, targeted modification of stress-related pathways through treatment with low amounts of highly bioactive substances offers an interesting alternative for agricultural use. The plant hormone CK is emerging as a promising new type of priming agents, the use of which appears to be closely linked to the control of the photosynthetic apparatus (Figure 1). Given the growing number of studies linking CK to defense or stress priming on the one hand and maintenance of photosynthesis on the other, more clear understanding on the mechanisms of a CK action on a photosynthetic and gas exchange machinery will be necessary for the more comprehensive understanding of CK-controlled stress protective responses. So far, mainly aromatic CKs BAP or KIN have been reported as priming agents with several possibilities of use. The application of natural CKs and many of their derivatives offers numerous advantages due to their negligible cytotoxicity to human cells and low risks to the environment resulting from their high biological activity in plants. However, although natural and some synthetic CKs show satisfactory results in terms of retarding senescence or maintaining balanced photosynthetic activity, they also show some negative effects on root development, and optimizing their effective use in field conditions remains a challenge. Therefore, new further SAR studies will be required to carefully promote the desirable traits of CK-based growth regulators to offer more practical future applications in sustainable agriculture.
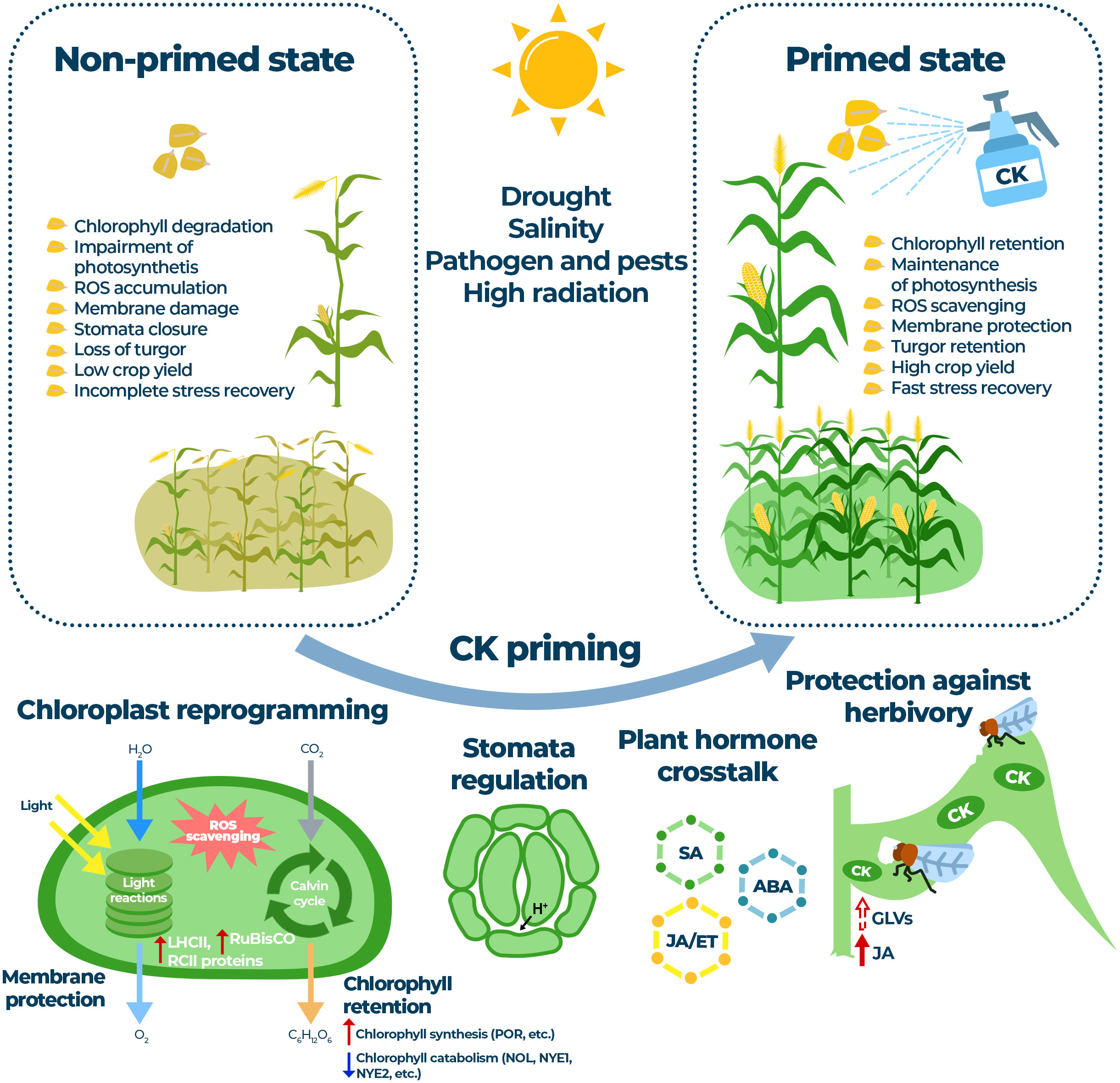
Figure 1 A simplified model showing the beneficial effects of cytokinin priming on photosynthesis and plant regulatory processes under stress conditions. Cytokinins can protect the light and dark reactions of photosynthesis by processes such as chlorophyll retention (upregulation of chlorophyll biosynthesis and downregulation of chlorophyll catabolism; cytokinin upregulated genes coding for POR, Protochlorophyllide oxidoreductase and downregulated genes coding for NOL, Chlorophyll b reductase; NYE1, Non-yellowing 1, and NYE2, Non-yellowing 2, are shown). Other cytokinin regulated processes include upregulation of LHCII and RCII components, upregulation of RuBisCO activity and activation of ROS scavenging system leading to membrane protection. Another important mechanism of action of cytokinins during stress and recovery is the desirable crosstalk of cytokinins with traditional plant stress hormones, potentially including volatile substances (GLVs). During herbivory, so-called “green islands” with elevated levels of CKs are formed; crosstalk mediated by volatile compounds and other phytohormones can initiate defense priming. Thus, the application of cytokinins can regulate plant response to various abiotic and biotic stresses, leading to balanced photosynthetic function, improved stress tolerance and increased crop yield.
Author contributions
MH and VN wrote the original draft of the manuscript. VN designed the figure with help of OP. OP and VN conceived the idea and made the final adjustments. All authors contributed to the article and approved the submitted version.
Funding
The work was supported by an ERDF project entitled “Development of Pre-Applied Research in Nanotechnology and Biotechnology” (No. CZ.02.1.01/0.0/0.0/17_048/0007323).
Acknowledgments
The authors wish to thank Kateřina Škrlová for her help with the graphic works and Jana Horáková for the language editing.
Conflict of interest
The authors declare that the research was conducted in the absence of any commercial or financial relationships that could be construed as a potential conflict of interest.
Publisher’s note
All claims expressed in this article are solely those of the authors and do not necessarily represent those of their affiliated organizations, or those of the publisher, the editors and the reviewers. Any product that may be evaluated in this article, or claim that may be made by its manufacturer, is not guaranteed or endorsed by the publisher.
References
Abhinandan, K., Skori, L., Stanic, M., Hickerson, N. M. N., Jamshed, M., Samuel, M. A. (2018). Abiotic stress signaling in wheat - an inclusive overview of hormonal interactions during abiotic stress responses in wheat. Front. Plant Sci. 9. doi: 10.3389/fpls.2018.00734
Alharby, H., Alzahrani, Y. M., Rady, M. (2020). Seeds pretreatment with zeatins or maize grain-derived organic biostimulant improved hormonal contents, polyamine gene expression, and salinity and drought tolerance of wheat. Int. J. Agric. Biol. 24, 714–724. doi: 10.17957/IJAB/15.1491
Amooaghaie, R., Tabatabaie, F. (2017). Osmopriming-induced salt tolerance during seed germination of alfalfa most likely mediates through H2O2 signaling and upregulation of heme oxygenase. Protoplasma 254, 1791–1803. doi: 10.1007/s00709-016-1069-5
Argueso, C. T., Ferreira, F. J., Epple, P., To, J. P. C., Hutchison, C. E., Schaller, G. E., et al. (2012). Two-component elements mediate interactions between cytokinin and salicylic acid in plant immunity. PloS Genet. 8, e1002448. doi: 10.1371/journal.pgen.1002448
Ariga, H., Katori, T., Tsuchimatsu, T., Hirase, T., Tajima, Y., Parker, J. E., et al. (2017). NLR locus-mediated trade-off between abiotic and biotic stress adaptation in arabidopsis. Nat. Plants 3, 1–8. doi: 10.1038/nplants.2017.72
Arun, M. N., Hebbar, S. S., Bhanuprakash, Senthivel, T., Nair, A. K., Padmavathi, G., et al. (2022). "Seed priming: The way forward to mitigate abiotic stress in crops." in Plant stress physiology - perspectives in agriculture. (IntechOpen). doi: 10.5772/intechopen.102033
Aswathi, K. P. R., Kalaji, H. M., Puthur, J. T. (2022). Seed priming of plants aiding in drought stress tolerance and faster recovery: a review. Plant Growth Regul. 97, 235–253. doi: 10.1007/s10725-021-00755-z
Avramova, Z. (2019). Defence-related priming and responses to recurring drought: Two manifestations of plant transcriptional memory mediated by the ABA and JA signalling pathways. Plant Cell Environ. 42, 983–997. doi: 10.1111/pce.13458
Badenoch-Jones, J., Parker, C. W., Letham, D. S., Singh, S. (1996). Effect of cytokinins supplied via the xylem at multiples of endogenous concentrations on transpiration and senescence in derooted seedlings of oat and wheat. Plant Cell Environ. 19, 504–516. doi: 10.1111/j.1365-3040.1996.tb00384.x
Barciszewski, J., Siboska, G., Rattan, S. I. S., Clark, B. F. C. (2000). Occurrence, biosynthesis and properties of kinetin (N6-furfuryladenine). Plant Growth Regul. 32, 257–265. doi: 10.1023/A:1010772421545
Bjornson, M., Benn, G., Song, X., Comai, L., Franz, A. K., Dandekar, A. M., et al. (2014). Distinct roles for mitogen-activated protein kinase signaling and CALMODULIN-BINDING TRANSCRIPTIONAL ACTIVATOR3 in regulating the peak time and amplitude of the plant general stress response. Plant Physiol. 166, 988–996. doi: 10.1104/pp.114.245944
Brenner, W. G., Schmülling, T. (2012). Transcript profiling of cytokinin action in arabidopsis roots and shoots discovers largely similar but also organ-specific responses. BMC Plant Biol. 12, 112. doi: 10.1186/1471-2229-12-112
Bruce, T. J. A., Matthes, M. C., Napier, J. A., Pickett, J. A. (2007). Stressful “memories” of plants: Evidence and possible mechanisms. Plant Sci. 173, 603–608. doi: 10.1016/j.plantsci.2007.09.002
Bryksová, M., Dabravolski, S., Kučerová, Z., Zavadil Kokáš, F., Špundová, M., Plíhalová, L., et al. (2020). Aromatic cytokinin arabinosides promote PAMP-like responses and positively regulate leaf longevity. ACS Chem. Biol. 15, 1949–1963. doi: 10.1021/acschembio.0c00306
Campos, M. L., Yoshida, Y., Major, I. T., de Oliveira Ferreira, D., Weraduwage, S. M., Froehlich, J. E., et al. (2016). Rewiring of jasmonate and phytochrome b signalling uncouples plant growth-defense tradeoffs. Nat. Commun. 7, 12570. doi: 10.1038/ncomms12570
Cary, A. J., Liu, W., Howell, S. H. (1995). Cytokinin action is coupled to ethylene in its effects on the inhibition of root and hypocotyl elongation in arabidopsis thaliana seedlings. Plant Physiol. 107, 1075–1082. doi: 10.1104/pp.107.4.1075
Chakrabarti, N., Mukherji, S. (2003). Alleviation of NaCl stress by pretreatment with phytohormones in vigna radiata. Biol. Plant 46, 589–594. doi: 10.1023/A:1024827931134
Cheikh, N., Jones, R. J. (1994). Disruption of maize kernel growth and development by heat stress (Role of Cytokinin/Abscisic acid balance). Plant Physiol. 106, 45–51. doi: 10.1104/pp.106.1.45
Chen, X., Ding, Y., Yang, Y., Song, C., Wang, B., Yang, S., et al. (2021). Protein kinases in plant responses to drought, salt, and cold stress. J. Integr. Plant Biol. 63, 53–78. doi: 10.1111/jipb.13061
Chernyad’ev, I. I. (2009). The protective action of cytokinins on the photosynthetic machinery and productivity of plants under stress (review). Appl. Biochem. Microbiol. 45, 351–362. doi: 10.1134/S0003683809040012
Chipilski, R., Moskova, I., Pencheva, A., Kocheva, K. (2021). Field priming with cytokinins enhances seed viability of wheat after low temperature storage. Plant Soil Environ. 67 (2021), 77–84. doi: 10.17221/524/2020-PSE
Choi, J., Choi, D., Lee, S., Ryu, C.-M., Hwang, I. (2011). Cytokinins and plant immunity: old foes or new friends? Trends Plant Sci. 16, 388–394. doi: 10.1016/j.tplants.2011.03.003
Choi, J., Huh, S. U., Kojima, M., Sakakibara, H., Paek, K.-H., Hwang, I. (2010). The cytokinin-activated transcription factor ARR2 promotes plant immunity via TGA3/NPR1-dependent salicylic acid signaling in arabidopsis. Dev. Cell 19, 284–295. doi: 10.1016/j.devcel.2010.07.011
Choi, W.-G., Toyota, M., Kim, S.-H., Hilleary, R., Gilroy, S. (2014). Salt stress-induced Ca2+ waves are associated with rapid, long-distance root-to-shoot signaling in plants. Proc. Natl. Acad. Sci. 111, 6497–6502. doi: 10.1073/pnas.1319955111
Conrath, U. (2006). Systemic acquired resistance. Plant Signal. Behav. 1, 179–184. doi: 10.4161/psb.1.4.3221
Conrath, U. (2011). Molecular aspects of defence priming. Trends Plant Sci. 16, 524–531. doi: 10.1016/j.tplants.2011.06.004
Conrath, U., Beckers, G. J. M., Flors, V., García-Agustín, P., Jakab, G., Mauch, F., et al. (2006). Priming: Getting ready for battle. Mol. Plant-Microbe Interactions 19, 1062–1071. doi: 10.1094/MPMI-19-1062
Conrath, U., Beckers, G. J. M., Langenbach, C. J. G., Jaskiewicz, M. R. (2015). Priming for enhanced defense. Annu. Rev. Phytopathol. 53, 97–119. doi: 10.1146/annurev-phyto-080614-120132
Cortleven, A., Leuendorf, J. E., Frank, M., Pezzetta, D., Bolt, S., Schmülling, T. (2019). Cytokinin action in response to abiotic and biotic stresses in plants. Plant Cell Environ. 42, 998–1018. doi: 10.1111/pce.13494
Cortleven, A., Schmülling, T. (2015). Regulation of chloroplast development and function by cytokinin. J. Exp. Bot. 66, 4999–5013. doi: 10.1093/jxb/erv132
Criado, M. V., Caputo, C., Roberts, I. N., Castro, M. A., Barneix, A. J. (2009). Cytokinin-induced changes of nitrogen remobilization and chloroplast ultrastructure in wheat (Triticum aestivum). J. Plant Physiol. 166, 1775–1785. doi: 10.1016/j.jplph.2009.05.007
Das, C., Sengupta, T., Chattopadhyay, S., Setua, M., Das, N. K., Saratchandra, B. (2002). Involvement of kinetin and spermidine in controlling salinity stress in mulberry (Morus alba l. cv. S1). Acta Physiol. Plant 24, 53–57. doi: 10.1007/s11738-002-0021-9
Daszkowska-Golec, A., Szarejko, I. (2013). Open or close the gate - stomata action under the control of phytohormones in drought stress conditions. Front. Plant Sci. 4. doi: 10.3389/fpls.2013.00138
de Moura, F. B., Vieira, M.R.da S., Simões, A.do N., Ferreira-Silva, S. L., de Souza, ,. C. A. V., de Souza, E. S., et al. (2018). Physiological effect of kinetin on the photosynthetic apparatus and antioxidant enzymes activities during production of anthurium. Hortic. Plant J. 4, 182–192. doi: 10.1016/j.hpj.2018.04.001
Dervinis, C., Frost, C. J., Lawrence, S. D., Novak, N. G., Davis, J. M. (2010). Cytokinin primes plant responses to wounding and reduces insect performance. J. Plant Growth Regul. 29, 289–296. doi: 10.1007/s00344-009-9135-2
de Zelicourt, A., Colcombet, J., Hirt, H. (2016). The role of MAPK modules and ABA during abiotic stress signaling. Trends Plant Sci. 21, 677–685. doi: 10.1016/j.tplants.2016.04.004
Engelberth, J., Alborn, H. T., Schmelz, E. A., Tumlinson, J. H. (2004). Airborne signals prime plants against insect herbivore attack. Proc. Natl. Acad. Sci. 101, 1781–1785. doi: 10.1073/pnas.0308037100
Engelberth, J., Viswanathan, S., Engelberth, M. J. (2011). Low concentrations of salicylic acid stimulate insect elicitor responses in zea mays seedlings. J. Chem. Ecol. 37, 263–266. doi: 10.1007/s10886-011-9926-3
Fan, Y., Ma, C., Huang, Z., Abid, M., Jiang, S., Dai, T., et al. (2018). Heat priming during early reproductive stages enhances thermo-tolerance to post-anthesis heat stress via improving photosynthesis and plant productivity in winter wheat (Triticum aestivum l.). Front. Plant Sci. 9. doi: 10.3389/fpls.2018.00805
Farber, M., Attia, Z., Weiss, D. (2016). Cytokinin activity increases stomatal density and transpiration rate in tomato. J. Exp. Bot. 67, 6351–6362. doi: 10.1093/jxb/erw398
Farmer, E. E., Ryan, C. A. (1990). Interplant communication: airborne methyl jasmonate induces synthesis of proteinase inhibitors in plant leaves. Proc. Natl. Acad. Sci. U. S. A. 87, 7713–7716. doi: 10.1073/pnas.87.19.7713
Fathy, M., Saad Eldin, S. M., Naseem, M., Dandekar, T., Othman, E. M. (2022). Cytokinins: Wide-spread signaling hormones from plants to humans with high medical potential. Nutrients 14, 1495. doi: 10.3390/nu14071495
Fragnière, C., Serrano, M., Abou-Mansour, E., Métraux, J.-P., L’Haridon, F. (2011). Salicylic acid and its location in response to biotic and abiotic stress. FEBS Lett. 585, 1847–1852. doi: 10.1016/j.febslet.2011.04.039
Frost, C. J., Mescher, M. C., Dervinis, C., Davis, J. M., Carlson, J. E., De Moraes, C. M. (2008). Priming defense genes and metabolites in hybrid poplar by the green leaf volatile cis-3-hexenyl acetate. New Phytol. 180, 722–734. doi: 10.1111/j.1469-8137.2008.02599.x
Giron, D., Kaiser, W., Imbault, N., Casas, J. (2007). Cytokinin-mediated leaf manipulation by a leafminer caterpillar. Biol. Lett. 3, 340–343. doi: 10.1098/rsbl.2007.0051
Gujjar, R. S., Banyen, P., Chuekong, W., Worakan, P., Roytrakul, S., Supaibulwatana, K. (2020). A synthetic cytokinin improves photosynthesis in rice under drought stress by modulating the abundance of proteins related to stomatal conductance, chlorophyll contents, and rubisco activity. Plants 9, 1106. doi: 10.3390/plants9091106
Haisel, D., Pospíšilová, J., Synková, H., Schnablová, R., Baťková, P. (2006). Effects of abscisic acid or benzyladenine on pigment contents, chlorophyll fluorescence, and chloroplast ultrastructure during water stress and after rehydration. Photosynthetica 44, 606–614. doi: 10.1007/s11099-006-0079-5
Hare, P. D., Cress, W. A., van Staden, J. (1997). The involvement of cytokinins in plant responses to environmental stress. Plant Growth Regul. 23, 79–103. doi: 10.1023/A:1005954525087
Hilker, M., Schmülling, T. (2019). Stress priming, memory, and signalling in plants. Plant Cell Environ. 42, 753–761. doi: 10.1111/pce.13526
Hirao, T., Okazawa, A., Harada, K., Kobayashi, A., Muranaka, T., Hirata, K. (2012). Green leaf volatiles enhance methyl jasmonate response in arabidopsis. J. Biosci. Bioeng. 114, 540–545. doi: 10.1016/j.jbiosc.2012.06.010
Holeski, L. M., Jander, G., Agrawal, A. A. (2012). Transgenerational defense induction and epigenetic inheritance in plants. Trends Ecol. Evol. 27, 618–626. doi: 10.1016/j.tree.2012.07.011
Hönig, M., Plíhalová, L., Husičková, A., Nisler, J., Doležal, K. (2018). Role of cytokinins in senescence, antioxidant defence and photosynthesis. Int. J. Mol. Sci. 19, 4045. doi: 10.3390/ijms19124045
Hu, L., Wang, Z., Huang, B. (2012). Growth and physiological recovery of Kentucky bluegrass from drought stress as affected by a synthetic cytokinin 6-benzylaminopurine. Crop Sci. 52, 2332–2340. doi: 10.2135/cropsci2012.02.0106
Iqbal, M., Ashraf, M. (2005). Presowing seed treatment with cytokinins and its effect on growth, photosynthetic rate, ionic levels and yield of two wheat cultivars differing in salt tolerance. J. Integr. Plant Biol. 47, 1315–1325. doi: 10.1111/j.1744-7909.2005.00163.x
Iqbal, M., Ashraf, M., Jamil, A. (2006). Seed enhancement with cytokinins: changes in growth and grain yield in salt stressed wheat plants. Plant Growth Regul. 50, 29–39. doi: 10.1007/s10725-006-9123-5
Islam, M. R., Islam, M. S., Akter, N., Mohi-Ud-Din, M., Mostofa, M. G. (2022b). Foliar application of cytokinin modulates gas exchange features, water relation and biochemical responses to improve growth performance of maize under drought stress. Phyton 91, 633–649. doi: 10.32604/phyton.2022.018074
Islam, M. R., Rahman, M. M., Mohi-Ud-Din, M., Akter, M., Zaman, E., Keya, S. S., et al. (2022a). Cytokinin and gibberellic acid-mediated waterlogging tolerance of mungbean (Vigna radiata l. wilczek). PeerJ 10, e12862. doi: 10.7717/peerj.12862
Janečková, H., Husičková, A., Lazár, D., Ferretti, U., Pospíšil, P., Špundová, M. (2019). Exogenous application of cytokinin during dark senescence eliminates the acceleration of photosystem II impairment caused by chlorophyll b deficiency in barley. Plant Physiol. Biochem. 136, 43–51. doi: 10.1016/j.plaphy.2019.01.005
Ji, N., Wang, J., Zuo, X., Li, Y., Li, M., Wang, K., et al. (2021). PpWRKY45 is involved in methyl jasmonate primed disease resistance by enhancing the expression of jasmonate acid biosynthetic and pathogenesis-related genes of peach fruit. Postharvest Biol. Technol. 172, 111390. doi: 10.1016/j.postharvbio.2020.111390
Johnson, R., Puthur, J. T. (2022). Biostimulant priming in oryza sativa: a novel approach to reprogram the functional biology under nutrient-deficient soil. Cereal Res. Commun. 50, 45–52. doi: 10.1007/s42976-021-00150-4
Kang, N. Y., Cho, C., Kim, N. Y., Kim, J. (2012). Cytokinin receptor-dependent and receptor-independent pathways in the dehydration response of arabidopsis thaliana. J. Plant Physiol. 169, 1382–1391. doi: 10.1016/j.jplph.2012.05.007
Kauss, H., Jeblick, W. (1995). Pretreatment of parsley suspension cultures with salicylic acid enhances spontaneous and elicited production of H2O2. Plant Physiol. 108, 1171–1178. doi: 10.1104/pp.108.3.1171
Kerchev, P., van der Meer, T., Sujeeth, N., Verlee, A., Stevens, C. V., Van Breusegem, F., et al. (2020). Molecular priming as an approach to induce tolerance against abiotic and oxidative stresses in crop plants. Biotechnol. Adv. 40, 107503. doi: 10.1016/j.biotechadv.2019.107503
Koprna, R., De Diego, N., Dundálková, L., Spíchal, L. (2016). Use of cytokinins as agrochemicals. Bioorg. Med. Chem. 24, 484–492. doi: 10.1016/j.bmc.2015.12.022
Kučerová, Z., Rác, M., Mikulík, J., Plíhal, O., Pospíšil, P., Bryksová, M., et al. (2020). The anti-senescence activity of cytokinin arabinosides in wheat and arabidopsis is negatively correlated with ethylene production. Int. J. Mol. Sci. 21, 8109. doi: 10.3390/ijms21218109
Kusnetsov, V., Herrmann, R. G., Kulaeva, O. N., Oelmüller, R. (1998). Cytokinin stimulates and abscisic acid inhibits greening of etiolated lupinus luteus cotyledons by affecting the expression of the light-sensitive protochlorophyllide oxidoreductase. Mol. Gen. Genet. MGG 259, 21–28. doi: 10.1007/PL00008626
Lau, S.-E., Teo, W. F. A., Teoh, E. Y., Tan, B. C. (2022). Microbiome engineering and plant biostimulants for sustainable crop improvement and mitigation of biotic and abiotic stresses. Discovery Food 2, 9. doi: 10.1007/s44187-022-00009-5
Lazár, D. (2015). Parameters of photosynthetic energy partitioning. J. Plant Physiol. 175, 131–147. doi: 10.1016/j.jplph.2014.10.021
Lemmens, E., Deleu, L. J., De Brier, N., De Man, W. L., De Proft, M., Prinsen, E., et al. (2019). The impact of hydro-priming and osmo-priming on seedling characteristics, plant hormone concentrations, activity of selected hydrolytic enzymes, and cell wall and phytate hydrolysis in sprouted wheat (Triticum aestivum l.). ACS Omega 4, 22089–22100. doi: 10.1021/acsomega.9b03210
Li, X., Xu, K. (2014). Effects of exogenous hormones on leaf photosynthesis of panax ginseng. Photosynthetica 52, 152–156. doi: 10.1007/s11099-014-0005-1
Li, C.-H., Yu, N., Jiang, S.-M., Shangguan, X.-X., Wang, L.-J., Chen, X.-Y. (2008). Down-regulation of s-adenosyl-L: -homocysteine hydrolase reveals a role of cytokinin in promoting transmethylation reactions. Planta 228, 125–136. doi: 10.1007/s00425-008-0724-2
Mangena, P. (2020). Role of benzyladenine seed priming on growth and physiological and biochemical response of soybean plants grown under high salinity stress condition. Int. J. Agron. 2020, e8847098. doi: 10.1155/2020/8847098
Masuta, C., Tanaka, H., Uehara, K., Kuwata, S., Koiwai, A., Noma, M. (1995). Broad resistance to plant viruses in transgenic plants conferred by antisense inhibition of a host gene essential in s-adenosylmethionine-dependent transmethylation reactions. Proc. Natl. Acad. Sci. U. S. A. 92, 6117–6121. doi: 10.1073/pnas.92.13.6117
Mauch-Mani, B., Baccelli, I., Luna, E., Flors, V. (2017). Defense priming: An adaptive part of induced resistance. Annu. Rev. Plant Biol. 68, 485–512. doi: 10.1146/annurev-arplant-042916-041132
Mik, V., Szüčová, L., Šmehilová, M., Zatloukal, M., Doležal, K., Nisler, J., et al. (2011). N9-substituted derivatives of kinetin: Effective anti-senescence agents. Phytochemistry 72, 821–831. doi: 10.1016/j.phytochem.2011.02.002
Mishina, T. E., Zeier, J. (2007). Pathogen-associated molecular pattern recognition rather than development of tissue necrosis contributes to bacterial induction of systemic acquired resistance in arabidopsis. Plant J. Cell Mol. Biol. 50, 500–513. doi: 10.1111/j.1365-313X.2007.03067.x
Mohammadi, H., Janmohammadi, M., Sabaghnia, N. (2015). Chlorophyll fluorescence response of wheat to exogenous application of growth regulators under terminal drought stress. Ann. Univ. Mariae Curie-Sklodowska Sect. C – Biol. 70, 13. doi: 10.17951/c.2015.70.1.13
Moradi, F., Ismail, A. M. (2007). Responses of photosynthesis, chlorophyll fluorescence and ROS-scavenging systems to salt stress during seedling and reproductive stages in rice. Ann. Bot. 99, 1161–1173. doi: 10.1093/aob/mcm052
Müller, M., Munné-Bosch, S. (2021). Hormonal impact on photosynthesis and photoprotection in plants. Plant Physiol. 185, 1500–1522. doi: 10.1093/plphys/kiaa119
Mur, L. A. J., Naylor, G., Warner, S. A. J., Sugars, J. M., White, R. F., Draper, J. (1996). Salicylic acid potentiates defence gene expression in tissue exhibiting acquired resistance to pathogen attack. Plant J. 9, 559–571. doi: 10.1046/j.1365-313X.1996.09040559.x
Naeem, M. S., Jin, Z. L., Wan, G. L., Liu, D., Liu, H. B., Yoneyama, K., et al. (2010). 5-aminolevulinic acid improves photosynthetic gas exchange capacity and ion uptake under salinity stress in oilseed rape (Brassica napus l.). Plant Soil 332, 405–415. doi: 10.1007/s11104-010-0306-5
Nawaz, A., Amjad, M., Khan, S. M., Afzal, I., Ahmed, T., Iqbal, Q., et al. (2013). Tomato seed invigoration with cytokinins. JAPS J. Anim. Plant Sci. 23, 121–128.
Nisler, J., Zatloukal, M., Spichal, L., Koprna, R., Dolezal, K., Strnad, M. (2015). 1,2,3-thiadiazol-5yl-urea derivatives, use thereof for regulating plant senescence and preparations containing these derivatives. U. S. Patent 9,993,002.
Oh, M.-H., Kim, J.-H., Zulfugarov, I. S., Moon, Y.-H., Rhew, T.-H., Lee, C.-H. (2005). Effects of benzyladenine and abscisic acid on the disassembly process of photosystems in anArabidopsis delayed-senescence mutant,ore9. J. Plant Biol. 48, 170–177. doi: 10.1007/BF03030405
Onkokesung, N., Baldwin, I. T., Gális, I. (2010). The role of jasmonic acid and ethylene crosstalk in direct defense of nicotiana attenuata plants against chewing herbivores. Plant Signal. Behav. 5, 1305–1307. doi: 10.4161/psb.5.10.13124
Pharmawati, M., Billington, T., Gehring, C. A. (1998). Stomatal guard cell responses to kinetin and natriuretic peptides are cGMP-dependent. Cell. Mol. Life Sci. CMLS 54, 272–276. doi: 10.1007/s000180050149
Pieterse, C. M. J., Leon-Reyes, A., van der Ent, S., Van Wees, S. C. M. (2009). Networking by small-molecule hormones in plant immunity. Nat. Chem. Biol. 5, 308–316. doi: 10.1038/nchembio.164
Pieterse, C. M. J., Zamioudis, C., Berendsen, R. L., Weller, D. M., Van Wees, S. C. M., Bakker, P. A. H. M. (2014). Induced systemic resistance by beneficial microbes. Annu. Rev. Phytopathol. 52, 347–375. doi: 10.1146/annurev-phyto-082712-102340
Plíhalová, L., Vylíčilová, H., Doležal, K., Zahajská, L., Zatloukal, M., Strnad, M. (2016). Synthesis of aromatic cytokinins for plant biotechnology. New Biotechnol. 33, 614–624. doi: 10.1016/j.nbt.2015.11.009
Pospíšilová, J. (2003). Interaction of cytokinins and abscisic acid during regulation of stomatal opening in bean leaves. Photosynthetica 41, 49–56. doi: 10.1023/A:1025852210937
Reed, R. C., Bradford, K. J., Khanday, I. (2022). Seed germination and vigor: Ensuring crop sustainability in a changing climate. Heredity 128, 450–459. doi: 10.1038/s41437-022-00497-2
Roháček, K. (2002). Chlorophyll fluorescence parameters: The definitions, photosynthetic meaning, and mutual relationships. Photosynthetica 40, 13–29. doi: 10.1023/A:1020125719386
Roser, M., Ritchie, H., Ortiz-Ospina, E. (2013). World population growth. In: Our world data. Available at: https://ourworldindata.org/world-population-growth (Accessed September 11, 2020).
Schäfer, M., Meza-Canales, I. D., Brütting, C., Baldwin, I. T., Meldau, S. (2015). Cytokinin concentrations and CHASE-DOMAIN CONTAINING HIS KINASE 2 (NaCHK2)- and NaCHK3-mediated perception modulate herbivory-induced defense signaling and defenses in nicotiana attenuata. New Phytol. 207, 645–658. doi: 10.1111/nph.13404
Shao, R., Wang, K., Shangguan, Z. (2010). Cytokinin-induced photosynthetic adaptability of zea mays l. to drought stress associated with nitric oxide signal: Probed by ESR spectroscopy and fast OJIP fluorescence rise. J. Plant Physiol. 167, 472–479. doi: 10.1016/j.jplph.2009.10.020
Singewar, K., Fladung, M., Robischon, M. (2021). Methyl salicylate as a signaling compound that contributes to forest ecosystem stability. Trees 35, 1755–1769. doi: 10.1007/s00468-021-02191-y
Sobol, S., Chayut, N., Nave, N., Kafle, D., Hegele, M., Kaminetsky, R., et al. (2014). Genetic variation in yield under hot ambient temperatures spotlights a role for cytokinin in protection of developing floral primordia. Plant Cell Environ. 37, 643–657. doi: 10.1111/pce.12184
Song, G. C., Ryu, C.-M. (2018). Evidence for volatile memory in plants: Boosting defence priming through the recurrent application of plant volatiles. Mol. Cells 41, 724–732. doi: 10.14348/molcells.2018.0104
Song, X.-G., She, X.-P., He, J.-M., Huang, C., Song, T., Song, X.-G., et al. (2006). Cytokinin- and auxin-induced stomatal opening involves a decrease in levels of hydrogen peroxide in guard cells of vicia faba. Funct. Plant Biol. 33, 573–583. doi: 10.1071/FP05232
Sorrentino, M., De Diego, N., Ugena, L., Spíchal, L., Lucini, L., Miras-Moreno, B., et al. (2021). Seed priming with protein hydrolysates improves arabidopsis growth and stress tolerance to abiotic stresses. Front. Plant Sci. 12. doi: 10.3389/fpls.2021.626301
Tanaka, Y., Sano, T., Tamaoki, M., Nakajima, N., Kondo, N., Hasezawa, S. (2006). Cytokinin and auxin inhibit abscisic acid-induced stomatal closure by enhancing ethylene production in arabidopsis. J. Exp. Bot. 57, 2259–2266. doi: 10.1093/jxb/erj193
Thomashow, M. F. (2010). Molecular basis of plant cold acclimation: Insights gained from studying the CBF cold response pathway. Plant Physiol. 154, 571–577. doi: 10.1104/pp.110.161794
Ton, J., Mauch-Mani, B. (2004). β-amino-butyric acid-induced resistance against necrotrophic pathogens is based on ABA-dependent priming for callose. Plant J. 38, 119–130. doi: 10.1111/j.1365-313X.2004.02028.x
Tsuda, K., Mine, A., Bethke, G., Igarashi, D., Botanga, C. J., Tsuda, Y., et al. (2013). Dual regulation of gene expression mediated by extended MAPK activation and salicylic acid contributes to robust innate immunity in arabidopsis thaliana. PloS Genet. 9, e1004015. doi: 10.1371/journal.pgen.1004015
Vedenicheva, N., Futorna, O., Shcherbatyuk, M., Kosakivska, I. (2022). Effect of seed priming with zeatin on secale cereale l. growth and cytokinins homeostasis under hyperthermia. J. Crop Improv. 36, 656–674. doi: 10.1080/15427528.2021.2000909
Vincent, C., Rowland, D., Schaffer, B., Bassil, E., Racette, K., Zurweller, B. (2020). Primed acclimation: A physiological process offers a strategy for more resilient and irrigation-efficient crop production. Plant Sci. 295, 110240. doi: 10.1016/j.plantsci.2019.110240
von Caemmerer, S., Farquhar, G. D. (1981). Some relationships between the biochemistry of photosynthesis and the gas exchange of leaves. Planta 153, 376–387. doi: 10.1007/BF00384257
Vylíčilová, H., Husičková, A., Spíchal, L., Srovnal, J., Doležal, K., Plíhal, O., et al. (2016). C2-substituted aromatic cytokinin sugar conjugates delay the onset of senescence by maintaining the activity of the photosynthetic apparatus. Phytochemistry 122, 22–33. doi: 10.1016/j.phytochem.2015.12.001
Wang, K., Jin, P., Han, L., Shang, H., Tang, S., Rui, H., et al. (2014). Methyl jasmonate induces resistance against penicillium citrinum in Chinese bayberry by priming of defense responses. Postharvest Biol. Technol. 98, 90–97. doi: 10.1016/j.postharvbio.2014.07.009
Wani, S. H., Kumar, V., Shriram, V., Sah, S. K. (2016). Phytohormones and their metabolic engineering for abiotic stress tolerance in crop plants. Crop J. 4, 162–176. doi: 10.1016/j.cj.2016.01.010
Wu, C., Cui, K., Wang, W., Li, Q., Fahad, S., Hu, Q., et al. (2016). Heat-induced phytohormone changes are associated with disrupted early reproductive development and reduced yield in rice. Sci. Rep. 6, 34978. doi: 10.1038/srep34978
Wu, C., Cui, K., Wang, W., Li, Q., Fahad, S., Hu, Q., et al. (2017). Heat-induced cytokinin transportation and degradation are associated with reduced panicle cytokinin expression and fewer spikelets per panicle in rice. Front. Plant Sci. 8. doi: 10.3389/fpls.2017.00371
Wu, A., Hammer, G. L., Doherty, A., von Caemmerer, S., Farquhar, G. D. (2019). Quantifying impacts of enhancing photosynthesis on crop yield. Nat. Plants 5, 380–388. doi: 10.1038/s41477-019-0398-8
Wu, L.-Y., Shang, G.-D., Wang, F.-X., Gao, J., Wan, M.-C., Xu, Z.-G., et al. (2022). Dynamic chromatin state profiling reveals regulatory roles of auxin and cytokinin in shoot regeneration. Dev. Cell 57, 526–542.e7. doi: 10.1016/j.devcel.2021.12.019
Wu, X., Zhu, Z., Li, X., Zha, D. (2012). Effects of cytokinin on photosynthetic gas exchange, chlorophyll fluorescence parameters and antioxidative system in seedlings of eggplant (Solanum melongena l.) under salinity stress. Acta Physiol. Plant 34, 2105–2114. doi: 10.1007/s11738-012-1010-2
Yakhin, O. I., Lubyanov, A. A., Yakhin, I. A., Brown, P. H. (2016). Biostimulants in plant science: A global perspective. Front. Plant Sci. 7. doi: 10.3389/fpls.2016.02049
Yang, D. Q., Luo, Y. L., Dong, W. H., Yin, Y. P., Li, Y., Wang, Z. L. (2018). Response of photosystem II performance and antioxidant enzyme activities in stay-green wheat to cytokinin. Photosynthetica 56, 567–577. doi: 10.1007/s11099-017-0708-1
Zatloukal, M., Plihalova, L., Klaskova, J., Spichal, L., Koprna, R., Dolezal, K., et al. (2015). Substituted 6-anilino-9-heterocyclylpurine derivatives for inhibition of plant stress. U. S. Patent 10,662,194.
Zavaleta-Mancera, H. A., López-Delgado, H., Loza-Tavera, H., Mora-Herrera, M., Trevilla-García, C., Vargas-Suárez, M., et al. (2007). Cytokinin promotes catalase and ascorbate peroxidase activities and preserves the chloroplast integrity during dark-senescence. J. Plant Physiol. 164, 1572–1582. doi: 10.1016/j.jplph.2007.02.003
Zdarska, M., Dobisová, T., Gelová, Z., Pernisová, M., Dabravolski, S., Hejátko, J. (2015). Illuminating light, cytokinin, and ethylene signalling crosstalk in plant development. J. Exp. Bot. 66, 4913–4931. doi: 10.1093/jxb/erv261
Zhang, X., Liu, D., Gao, D., Zhao, W., Du, H., Qiu, Z., et al. (2022). Cytokinin confers brown planthopper resistance by elevating jasmonic acid pathway in rice. Int. J. Mol. Sci. 23, 5946. doi: 10.3390/ijms23115946
Zhao, M., Wang, L., Wang, J., Jin, J., Zhang, N., Lei, L., et al. (2020). Induction of priming by cold stress via inducible volatile cues in neighboring tea plants. J. Integr. Plant Biol. 62, 1461–1468. doi: 10.1111/jipb.12937
Keywords: priming, cytokinin, photosynthesis, stomata, ROS, stress, chlorophyll fluorescence
Citation: Hudeček M, Nožková V, Plíhalová L and Plíhal O (2023) Plant hormone cytokinin at the crossroads of stress priming and control of photosynthesis. Front. Plant Sci. 13:1103088. doi: 10.3389/fpls.2022.1103088
Received: 19 November 2022; Accepted: 29 December 2022;
Published: 18 January 2023.
Edited by:
Paromik Bhattacharyya, Institute of Himalayan Bioresource Technology (CSIR), IndiaReviewed by:
Marcelo Nogueira Do Amaral, Federal University of Pelotas, BrazilCopyright © 2023 Hudeček, Nožková, Plíhalová and Plíhal. This is an open-access article distributed under the terms of the Creative Commons Attribution License (CC BY). The use, distribution or reproduction in other forums is permitted, provided the original author(s) and the copyright owner(s) are credited and that the original publication in this journal is cited, in accordance with accepted academic practice. No use, distribution or reproduction is permitted which does not comply with these terms.
*Correspondence: Ondřej Plíhal, ondrej.plihal@upol.cz