- 1Department of Geology, National Museum of Natural Sciences (MNCN-CSIC), Madrid, Spain
- 2Laboratory of Fungal Genetics and Metabolism, Institute of Microbiology of the CAS, Prague, Czechia
- 3Department of Agrochemistry, Environmental Microbiology and Soil Conservation, Institute of Natural Resources and Agricultural Biology (IRNAS-CSIC), Seville, Spain
- 4Department of Biology and Geology, University of Almeria, Almeria, Spain
- 5Department of Geology, Geography and Environment, University of Alcala, Alcala de Henares, Spain
Castañar Cave is a clear example of an oligotrophic ecosystem with high hygrothermal stability both seasonal and interannual and the particularity of registering extraordinary levels of environmental radiation. These environmental conditions make the cave an ideal laboratory to evaluate both the responses of the subterranean environment to sudden changes in the matter and energy fluxes with the exterior and also any impact derived from its use as a tourist resource under a very restrictive access regime. In 2008, a fungal outbreak provoked by a vomit contaminated the sediments which were removed and subsequently treated with hydrogen peroxide. Fungal surveys were carried out in 2008 and 2009. The visits were resumed in 2014. Here, 12 years after the outbreak, we present an exhaustive study on the cave sediments in order to know the distribution of the different fungal taxa, as well as the prevalence and spatio-temporal evolution of the fungi caused by the vomit over the years under the conditions of relative isolation and high radiation that characterize this cave.
Introduction
Castañar Cave (Castañar de Ibor, Caceres, Spain) was discovered in 1967, declared Natural Monument in 1997 and opened to visits in 2003. The interest of this cavity lies in its great variety of speleothems formed mainly by aragonite and calcite of low magnesium (Alonso-Zarza et al., 2011) and in its very high concentrations of radon (222Rn) in air with annual average above 30 kBq/m3 (Lario et al., 2006; Garcia-Guinea et al., 2013). These values are much higher than the average 222Rn concentrations found in most caves studied around the world (0.5–8.3 kBq/m3; Cigna, 2005; Somlai et al., 2011). 222Rn was used as tracer for assessing ventilation of Castañar Cave because its variations depend only on the exchange of air with the exterior (Fernandez-Cortes et al., 2009). The slates hosting the cave contain uranium (39.2 ± 5.2 mg.kg−1; Garcia-Guinea et al., 2013), so that its disintegration to radon and the subsequently 222Rn exhalation from bedrock entails a continuous source of this gas to cave atmosphere. The weathering leakage processes of the bedrock also favor the remobilization of radionuclides via leaching and their later settlement into the cave environment associated to mineral phases of cave deposits (Garcia-Guinea et al., 2013). This favors maintaining the high 222Rn activity of cave air since there is a continuous regeneration of this gas due to the long-lived of these radionuclides of the radium radioactive decay chain.
The morning of August 26, 2008, on the cave walls and ground sediments appeared long, white fungal mycelia as the result of the vomit of a visitor, 40 h before. The vomit area was located at 17 m from the cave entrance and the ground sediments exhibited a widespread fungal colonization after the disturbance. In the following days, visitors stepped on the sediments colonized by the fungi, and detritus, mycelia and spores were distributed along the touristic trail, as evidenced by the presence of patches of fungal growth farther than the vomit area (Jurado et al., 2010). To help to control the outbreak, the entire area directly affected by vomiting was cleaned and subsequently treated with hydrogen peroxide and the visits were cancelled on September 11, 2008, 16 days after the spillage. In 2009, the cave was only visited by scientists and staff people for controlling the fungal outbreak. The cave was reopened in 2014 but the number of visitors/year was reduced from 1,500 to 1,600 in 2008, to an annual maximum of 450 visitors. Besides, since the cave re-opened, in order to minimize the entrance of external material, the visitors uses clean and uncontaminated suits and shoe covers.
In Castañar Cave, we had the opportunity to carry out extensive microbiological studies on 2008 and 2009, up to 12 months after the fungal outbreak, and again 12 years after the outbreak in a subsequent and exhaustive study carried out in 2020. Castañar Cave is a natural environment with high levels of ionizing radiation but also a low energy cave and high environmental stability throughout the annual cycle (Fernandez-Cortes et al., 2011) which make it more sensitive to the entry of matter and energy from outside. There are a few reports about the dominance of microbial communities in radioactive environments such as the International Space Station and Chernobyl reactor (Dadachova and Casadevall, 2008; Van Houdt et al., 2012; Romsdahl et al., 2018). These works give some examples of microbial species and melanized fungi with a high tolerance to radioactivity. However, research works in natural environments with high ionizing radiation and on the evolution of microbial populations in these environments are very scarce (Siasou et al., 2017).
The direct control that environmental conditions exert on the distribution of fungi in subterranean ecosystems has recently been demonstrated (Jurado et al., 2021; Sanchez-Moral et al., 2021). The objective of this study is to know the distribution of the different fungal taxa throughout Castañar Cave, as well as the prevalence and spatio-temporal evolution of the fungi caused by the vomit over the years under conditions of high isolation of the cave atmosphere from the outside and an outstanding radon activity.
Materials and Methods
Site
Castañar Cave (SW Spain, 39°37′40´´N, 5°24′59´´W, 590 m.a.s.l.) is currently located under a natural olive grove that grows in a temperate and semi-arid climate with a rainfall regime of less than 500 mm per year, long periods of drought in summer and maximum rains in autumn. Outside, the annual average temperature is 15.9°C and the average relative humidity is 62.5%.
The cave was developed by dissolution of dolomite strata interbedded in shales and greywackes of the Precambrian Age (Alonso-Zarza and Martin-Perez, 2008). Castañar Cave is a small karstic cavity with a cumulative length of 2,135 m, distributed in six main and wider halls (e.g., Sala Nevada) and some narrow galleries connecting them, with heights of less than 3 m in any case (Figure 1). The cave entrance is a unique vertical access, 9 m long over an area of 1.5 m2, with a quasi-hermetic door installed at the entrance. All these characteristics make Castañar a low energy cave with very high 222Rn gas concentration and high environmental stability throughout the annual cycle (Fernandez-Cortes et al., 2011).
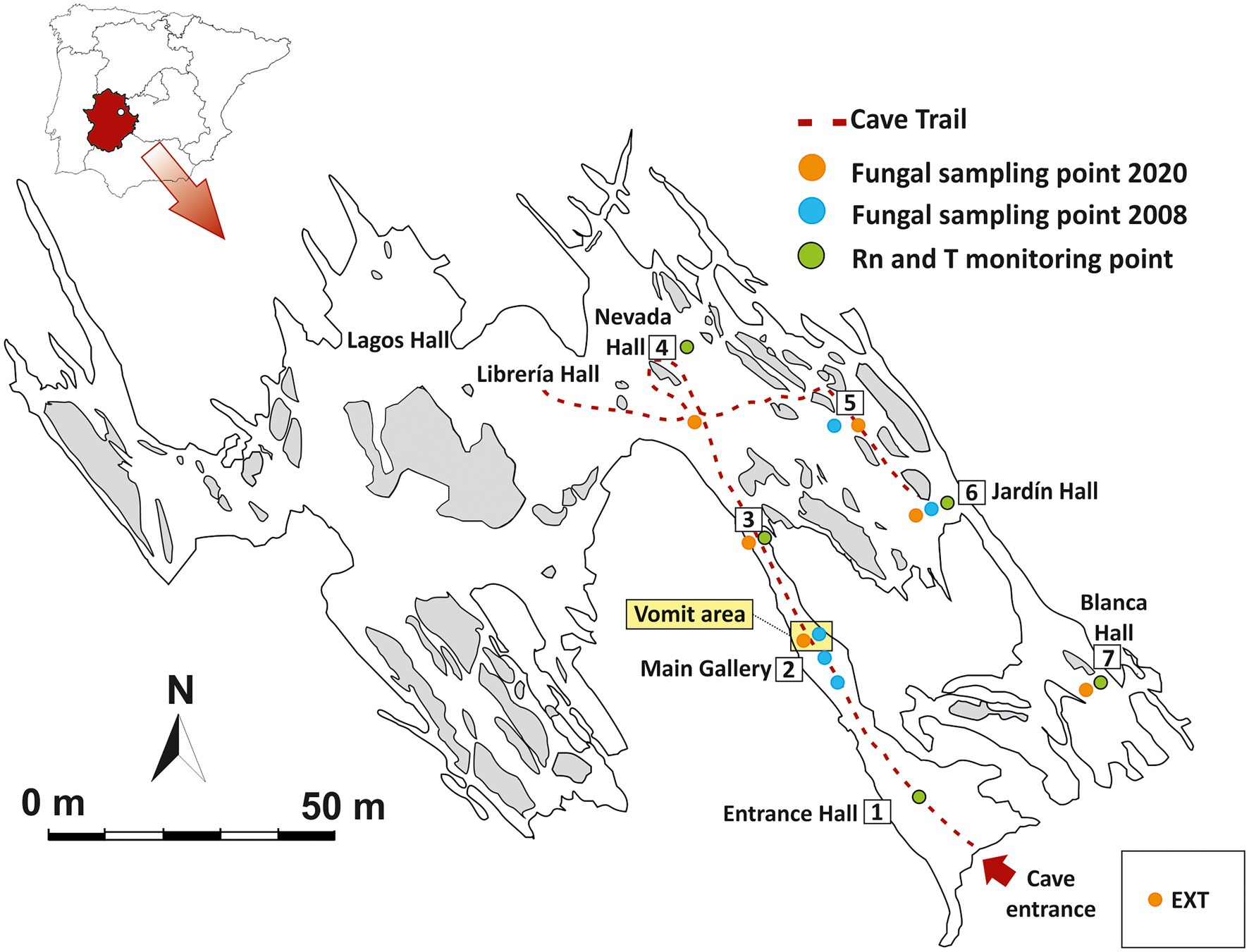
Figure 1. Map of Castañar Cave with location of sampling points, passive radon dosimeters and thermohygrometer recorders for environmental monitoring. EXT: Exterior.
Environmental Surveys
Variations in 222Rn concentration and air temperature are two key environmental parameters to determine the exchange rates of matter and energy and the main connection pathways between the exterior and the cavity. A set of five autonomous thermohygrometer recorders (Tinytag TGP-4500) and five nuclear track-etch passive detectors equipped with a solid state LR-115 type film (Kodak) were installed inside the cave, distributed according to the geomorphological characteristics of the subterranean galleries and the distance to the entrance (Figure 1). This monitoring network makes it possible to obtain monthly mean values of the 222Rn concentration of the subterranean air and a continuous thermo-hygrometric record at each point.
Microbiological Analysis
On December 3, 2020, seven sediment samples (approximately 3–4 cm depth) were collected in Castañar Cave (P1-P7) and one from the exterior soil (E) near the cave entrance (Figure 1). All samples were collected using sterile spatulas and immediately suspended in DNA/RNA Shield™ into sterile polypropylene tubes. Then, the samples were stored at 4°C, in the dark during transportation and were kept at −80°C until processing.
Total DNA was extracted from environmental samples using the QIAGEN Power Soil Kit, following the manufacturer’s instructions. Two independent DNA extractions were carried out for each sampling point, except in P1 where we only collected one sample. Extracted DNA was used as template for generating PCR-amplicons. The internal transcribed spacer 2 (ITS2) of the nuclear ribosomal DNA (about 400 bp) was amplified using the primers ITS86F (5 ‘GTGAATCATCGAATCTTTGAA 3′; Turenne et al., 1999) and ITS4 (5 ‘TCCTCCGCTTATTGATATGC 3′; White et al., 1990). Amplifications settings were as follows: 95°C for 5 min followed by 35 cycles consisting of 95°C for 30s, 49°C for 30 s, and 72°C for 30s and a 10-min elongation step at 72°C. PCR-amplicons were sequenced by high-throughput sequencing at AllGenetics Company (A Coruña, Spain) on the Illumina MiSeq platform using the paired-end reads 2 × 300 bp reagent kit, according to the manufacturer’s instructions. Extraction and PCR blanks were used.
To analyze fungal community composition, Raw fastq files were processed with QIIME2 (Bolyen et al., 2019). Briefly, DADA2 (Callahan et al., 2016) filtered the raw data according to the quality, generating an amplicon sequence variant (ASV) table. Then, taxonomic assignments were determined for ASVs using qiime2-feature-classifier classify-sklearn against the UNITE fungal ITS database (version 8.0; UNITE Community, 2019) and trained with Naive Bayes classifier on the full reference sequences. Taxa were also blasted (BlastN) against GenBank for fungal verification. All fungal names were reported according to the MycoBank Database. The sequencing data was further imported into the R environment 3.6.0 and processed using the phyloseq package (McMurdie and Holmes, 2013). Barplots and clustered heat maps by euclidean distance were prepared using ggplot and pheatmap packages to illustrate the composition of fungal communities in Castañar Cave. Alpha diversity was calculated using Shannon diversity index and Faith’s Phylogenetic Diversity, directly using QIIME2. The raw reads were deposited into the NCBI Sequence Read Archive (SRA) database under accession number PRJNA802044.
Results
Environmental Parameters
Figure 2 shows the annual mean values and the annual oscillation ranges of 222Rn concentration of the monitored area. The spatio-temporal distribution of tracer gases is directly related to morphology and cave air circulation. The results show that the entire cave has a very high level of natural radiation throughout the year, higher than 30 kBq/m3, ranging from 32,440 Bq/m3 in the Jardin area (P5-P6) to 34,940 Bq/m3 (P1-P7). The highest mean concentrations and the maximum variations (in red tones) are recorded in the area near the entrance (P1) and in Sala Blanca (P7) – Sala Final, the end of the cave. On the contrary, the most stable area throughout the year is Sala Nevada (P4) with an intra-annual variation of 19,942 Bq/m3.
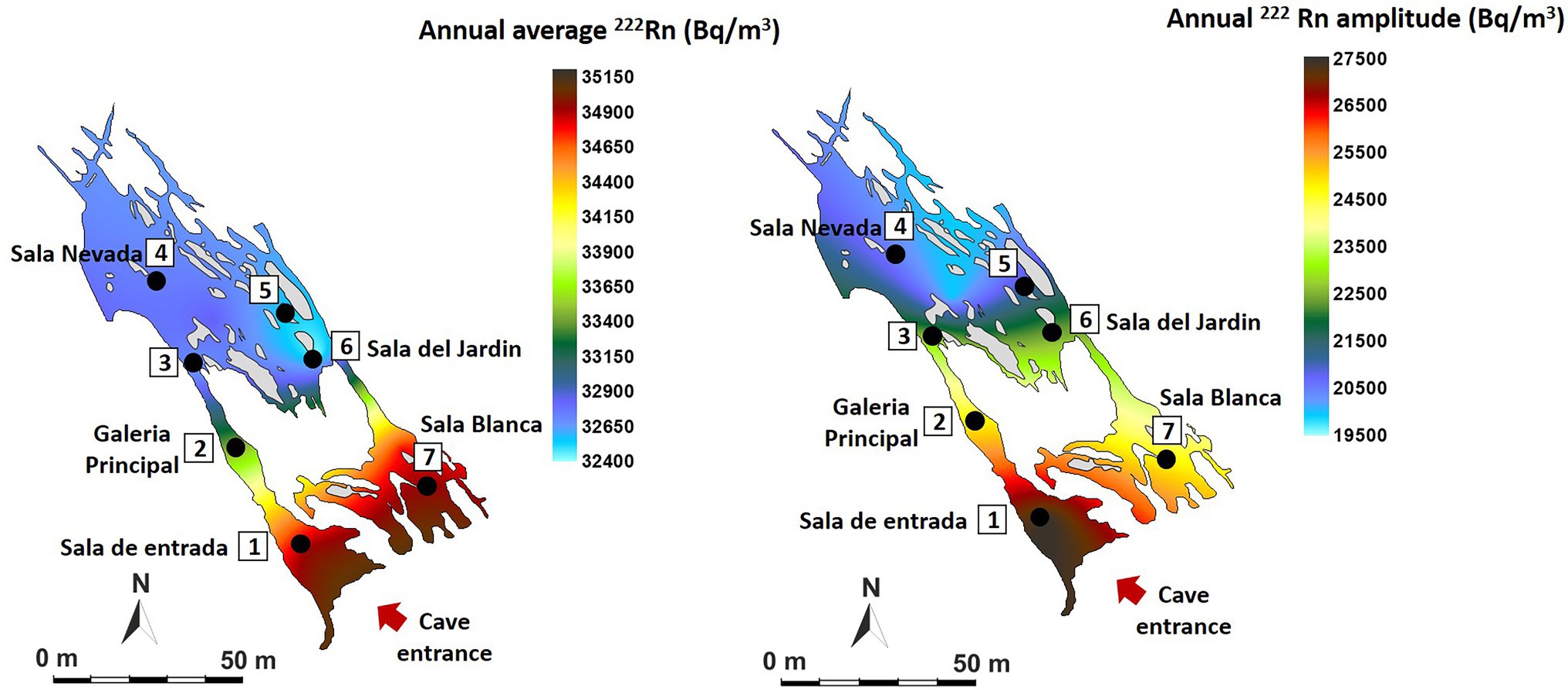
Figure 2. Spatial distribution in Castañar Cave of mean annual radon concentration and maximum amplitude of annual variation expressed in Bq/m3.
In the case of air temperature (Figure 3), the annual mean values range from 17.08°C in the internal zone (P5-P6) to 17.81°C in the areas near the entrance (P1). The data show the high environmental stability of the cave which annual variation ranges below 0.25°C at all measurement points except in the entrance area (P1, 0.71°C of annual amplitude, red tones), where the external direct influence is evident. These data indicate that the sectors close to the entrance show the highest rates of air renewal and consequently of matter and energy exchange with the outside atmosphere. On the contrary, the most stable area with the least energy exchange with the exterior is located in P5-P6 (Sala del Jardin, in light blue tones).
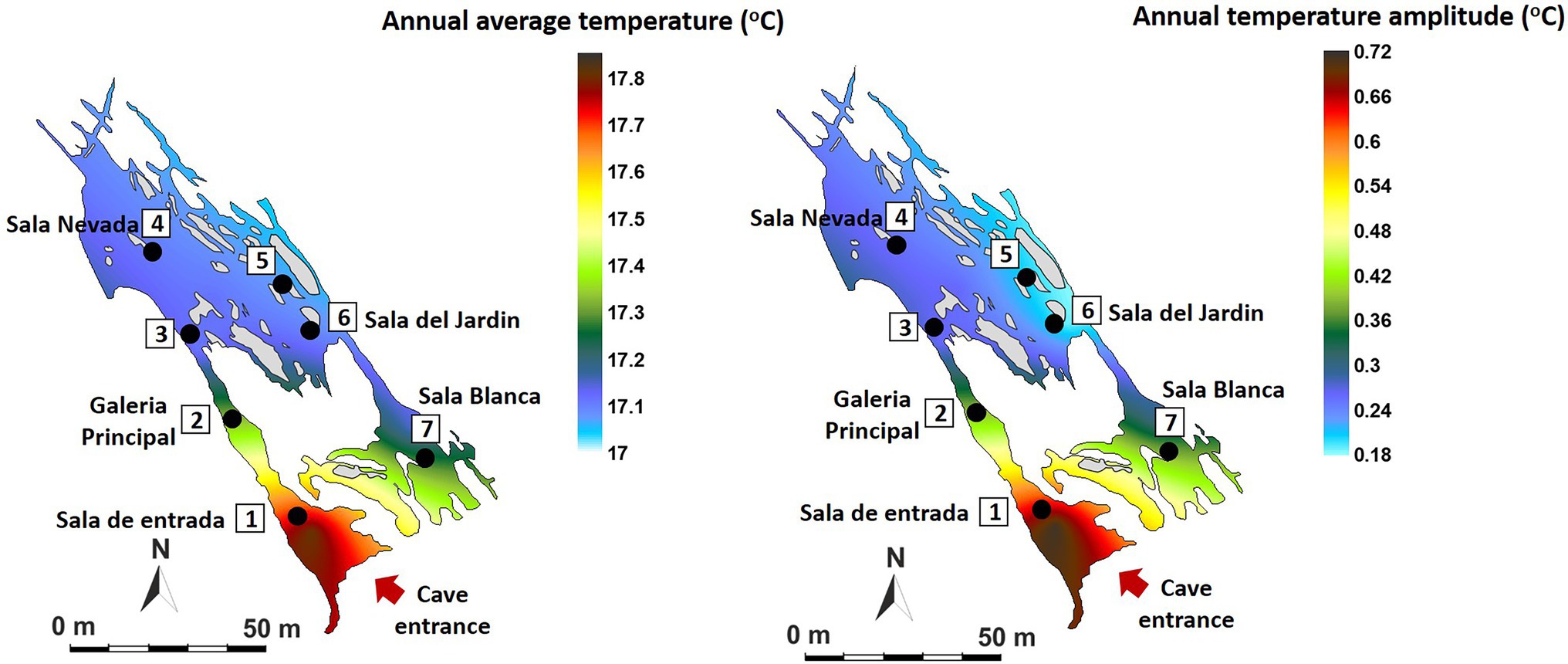
Figure 3. Spatial distribution in Castañar Cave of mean annual air temperature and maximum amplitude of annual variation expressed in degrees centigrade (°C).
Fungal Communities in 2020
Six samples from the cave sediments were taken in December 2020 along the touristic trail and one soil sample outside the cave, which were used for molecular studies. Sample P-3 was not studied.
Supplementary Table S1 shows the alpha diversity indices and how fungal diversity decreased in the cave environment when compared to the topsoil. Inside the cave, the lowest fungal diversity was observed in the internal zone (P5-P6), while the highest was found in Sala de Entrada, near the cave entrance. These data indicate that fungal diversity increased with the entry of external organic matter and only a few species survive in the most oligotrophic areas (P5-P6).
Figure 4 shows phyla distribution in the different samples. In general, there is an uneven distribution between the phyla Ascomycota and Basidiomycota, with a predominance of ascomycetes in the exterior soil (E) and the first part of the trail from Sala de Entrada (P1) to Sala Nevada (P4), which reversed in the last part of the trail, especially in P5 with a majority of Basidiomycota. At the end of the trail, Sala del Jardin (P6) and Sala Blanca (P7) the relative abundances of both phyla were equivalent. Other abundant phyla were Mortierellomycota in P4 and Glomeromycota in the soil (E). The quantities of other phyla were negligible, below 1%, except for Monoblepharomycota that reached 2.1% in the soil (E).
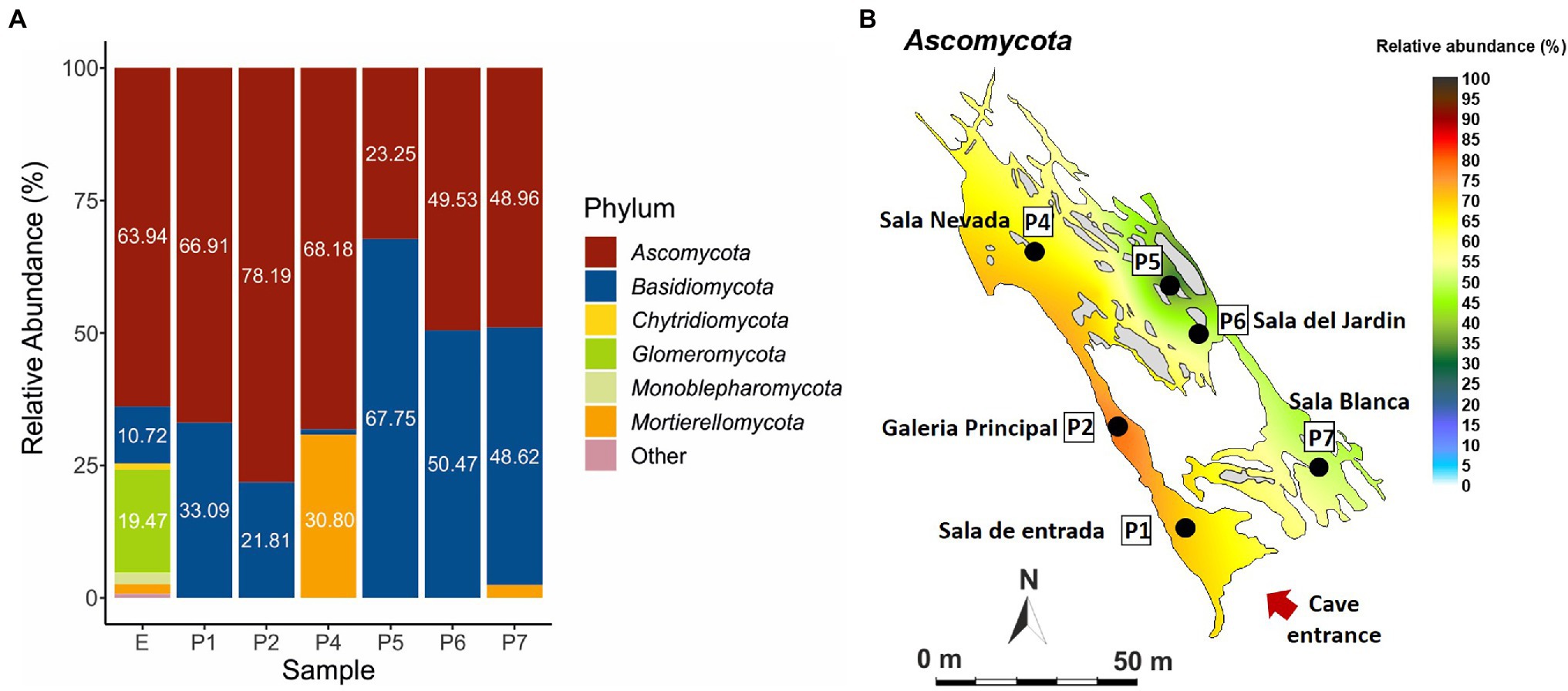
Figure 4. Fungal abundance at phylum level in Castañar Cave, in the December 2020 campaign. (A) Barplot illustrating fungal abundance at phylum level in P1-P7, and exterior soil E. Phyla with abundances below 1% are represented as Other. (B) Spatial distribution of Ascomycota in the December 2020 campaign.
Figure 5 and Supplementary Table S2 illustrate the relative abundance of major fungi (higher than 1% in at least one sample) at the species level. Thirty five fungi were identified at the species level, while 14 were only at genus level. The most abundant species was Candida parapsilosis followed by Sistotrema oblongisporum, Cephalotrichum microsporum, Cystobasidium slooffiae, Mortierella alpina, Omphalotus olearius, Neocosmospora solani (= Fusarium solani), Trichophyton ajelloi, Stereum hirsutum, and Malassezia globosa.
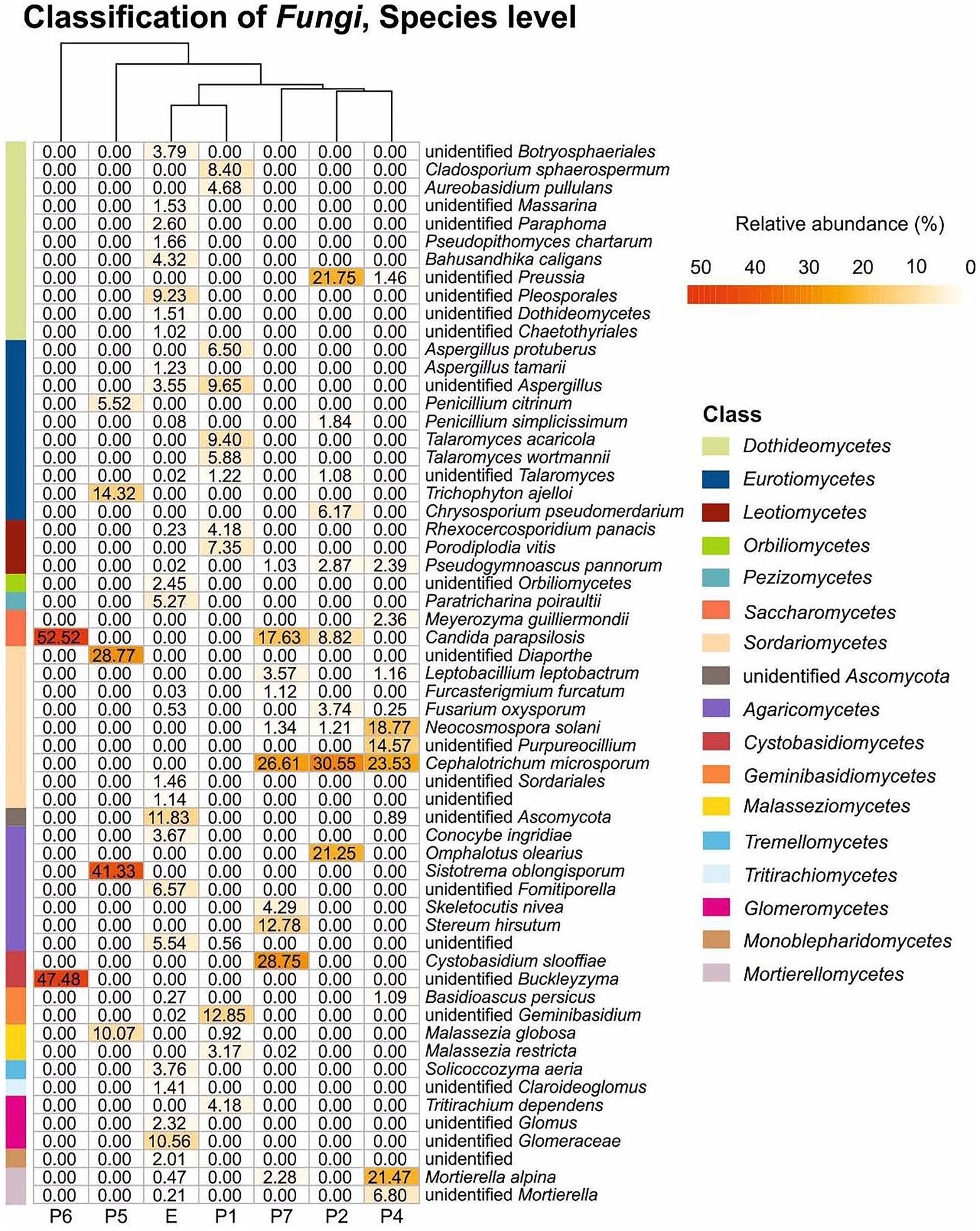
Figure 5. Fungal heat map in Castañar Cave samples. Scales bar show the relative abundance at species level, white squared represents the least abundant species and red the most abundant. Branching patterns on top of each heat map group sediment samples by shared species relative abundance patterns. Bars on the left and the legend on the right indicate the classification at the class level. The species with abundances below 1% are not included.
Other species identified, although with relative abundance below 5% were Pseudopithomyces chartarum, Bahusandhika caligans, Aspergillus tamari, Conocybe ingridiae, and Solicoccozyma aeria, which were only found in the soil (E), and Penicillium simplicissimum, Pseudogymnoascus pannorum, Meyerozyma guilliermondii, Leptobacillium leptobactrum, Fusarium oxysporum, Acremonium furcatum, Skeletocutis nivea, and Basidioascus persicus in different halls.
Among the unidentified members of genera and families were predominant Buckleyzyma, Diaporthe, Preussia, Purpureocillium, Glomeraceae and Mortierella. With relative abundance below 5% were members of the genera Massarina, Paraphoma, Aspergillus, Claroideoglomus and Glomus in the soil (E) and Talaromyces in the cave.
Discussion
Fungi in Castañar Cave 12 Years After a Fungal Outbreak
Only three fungi retrieved from previous surveys in sediments (2008–2009) were found again in the 2020 campaign: Neocosmospora solani, Fusarium oxysporum, and Mortierella alpina. It must be noticed that the comparison was made within isolates and NGS sequences, which could create some bias. Nevertheless, their presence in the cave despite the extensive cleaning of sediments carried after the spillage suggests that these three fungi are resistant and well adapted to the oligotrophic and high radiation conditions of the cave.
The occurrence of Neocosmospora solani was detected in three sediment samples (Galeria Principal, Sala Nevada and Sala Blanca) and seems to be a permanent inhabitant of Castañar Cave, present in all samplings since 2008. From a point of view of cave conservation, Neocosmospora solani is a dangerous fungus and has been widely reported in relation to cave outbreaks (Dupont et al., 2007; Bastian et al., 2009), and particularly in Castañar Cave (Jurado et al., 2010).
In some caves Fusarium oxysporum was associated with Neocosmospora solani (Jiang et al., 2017; Popkova and Mazina, 2019), as they are in Castañar Cave. Fusarium oxysporum is widely found as a plant pathogen, endophyte, and soil saprobe and was isolated from Chernobyl (Urbaniak et al., 2019).
Mortierella alpina and other Mortierella species were usually abundant in bat dung samples collected in caves (Degawa and Gams, 2004). Mortierella species were reported to be present in all of the 39 samples collected across five habitats, including sediments, weathered rocks, bat guano, drip waters, and air in Heshang Cave, China (Man et al., 2018). In addition to Fusarium, Mortierella species have been isolated from bat carcasses found in caves (Karunarathna et al., 2020; Nováková, 2021). In Castañar Cave no bats have been reported, but rodents, geckos and their feces were found. We have detected brushite (hydrated calcium phosphate) in the sediments, a mineral from guano-rich caves (Hill and Forti, 1997; Giurgiu and Tamas, 2013). However, brushite is also excreted by rats (Khan, 1998).
Regarding the most abundant taxa (>10%) found in 2020 (Figure 5) 17 taxa stand out. Candida parapsilosis was previously isolated from organs and viscera of bats captured in Brazilian urban forests (Ludwig et al., 2023). Other different and abundant Candida spp. were isolated from guano and intestinal contents of bats captured in Mexican caves (Ulloa et al., 2006). The occurrence in Castañar Cave is likely associated with the feces of rodents inhabiting the cave.
The genus Cephalotrichum seems to be dominant in some caves (Nováková, 2009; Nováková et al., 2018; Vanderwolf et al., 2019). Jiang et al. (2017) isolated 30 strains of Cephalotrichum from a Chinese cave, and three were described as new species. Cephalotrichum microsporum, a fungus not detected in 2008, was one of the most abundant in the 2020 sampling (Supplementary Table S2; Figure 5), and is a saprophytic fungus often reported from animal dung and soils (Heredia et al., 2018; Supplementary Tables S3, S4). However, its abundance represents a potential risk for the conservation of the cave. As well as Candida parapsilosis its presence could be associated with animal dung.
The connection from Sala Nevada to Sala del Jardin (P5) is characterized by the dominance of an unidentified species of Diaporthe, followed by Trichophyton ajelloi, and Penicillium citrinum, among ascomycetes. Diaporthe is a genus mainly composed of plant pathogens, responsible for diseases on a wide range of plants, in addition to endophytes and saprobes (Gomes et al., 2013). Diaporthe spp. have been isolated from the air in caves from China (Zhang et al., 2017), Brazil (Cunha et al., 2020), Spain (Sanchez-Moral et al., 2021), and from bats in Malaysian caves (Wasti et al., 2020). Trichophyton ajelloi (=Arthroderma uncinatum) is a geophilic dermatophyte that can cause infections in humans, with a preference for keratin-rich substrates (Zheng et al., 2020). Other Trichophyton spp. have been isolated from cave air (Nováková, 2009; Martin-Sanchez et al., 2014; Sanchez-Moral et al., 2021).
Conversely, in the connection from Sala Nevada to Sala del Jardin (P5) the basidiomycetes are composed of Sistotrema oblongisporum (41.33%) and Malassezia globosa (10.07%). Sistotrema spp. have been previously found in mines, always associated with timbers (Eslyn and Lombard, 1983; Held et al., 2020). The presence of these basidiomycetes in the cave could be related with a definite nutrient source, and the most common could be the presence of wood and/or branches fragments. Malassezia globosa is a cold-adapted yeast with the ability to survive in extreme conditions (Connell et al., 2014). This species requires lipids for growth and is common in human dandruff and seborrheic dermatitis and may be an indication of human or animal activity (Connell and Staudigel, 2013).
Sala del Jardin (P6) is characterized by the strong relative abundance of two yeasts, Candida parapsilosis (Ascomycota, Saccharomycetes) and an unidentified species of the genus Buckleyzyma (Basidiomycota, Cystobasidiomycetes), both covering 100% of the relative abundance. It has been reported that Candida parapsilosis and other yeasts are associated with basidiomycetes (Péter et al., 2017), as we found in Galeria Principal (P2) and Sala Nevada (P7). The genus Buckleyzyma comprises species previously placed in the genera Rhodotorula, Sporobolomyces and Bullera (Wang et al., 2016). Species of Buckleyzyma have been isolated from litter (Mašínová et al., 2017), plant leaves and soils (Li et al., 2020), as well as from floor and walls of a winery (Abdo et al., 2020). In most of these environments, Buckleyzyma was associated with Candida, Solicoccozyma, Malassezia, Meyerozyma, and other yeasts. Species of Buckleyzyma, Candida, Malassezia, and Meyerozyma were found in Castañar Cave and Solicoccozyma in the soil outside the cave.
The genus Preussia (Pleosporales, Sporormiaceae), abundant in the vomit area, Galeria Principal (P2), is widespread on different types of animal dung, and soil, decayed wood, and plant debris. Gonzalez-Menendez et al. (2017) reported that 28 out of 29 species of Preussia from the Iberian Peninsula were isolated from dung.
Omphalotus olearius (basidiomycetes) and Chrysosporium pseudomerdarium (ascomycetes) only appeared in Galeria Principal (P2). The habitat of Omphalotus olearius is olive trees (Castro et al., 2011) in accordance with the extensive olive grove under which the cavity is located. This basidiomycete species clearly point to an origin outside the cave and their transport inside, as it was found near the cave entrance. Chrysosporium pseudomerdarium was isolated from soils, caves and bat guano (Nagai et al., 1998; Larcher et al., 2003; Saxena et al., 2004). Most species of Chrysosporium are keratinolytic (Bohacz, 2017).
A few basidiomycete species appeared exclusively in Sala Blanca (P7): Cystobasidium slooffiae (28.75%), Stereum hirsutum (12.78%) and Skeletocutis nivea (4.29%). Sistotrema oblongisporum is a corticioid fungus, as well as Stereum hirsutum and Skeletocutis nivea. These fungi are wood-rotting species, common in forest on dead branches and logs (Korhonen et al., 2018). Conversely, Cystobasidium slooffiae, previous known as Rhodotorula slooffiae, was found on bats in Australian caves (Holz et al., 2018). Sala Blanca (P7), at the end of the trail, is rarely visited but the environmental data indicated an external direct influence. The occurrence of these basidiomycetes could be associated to the connection of Sala Blanca with the exterior. Another basidiomycete, an unidentified Geminibasidium occurred in Sala de Entrada with relative abundance of 12.85%, but only 0.02% in the exterior soil. The species of this genus have been isolated from forest soils and rhizosphere (Nguyen et al., 2013; Ren et al., 2021).
Other fungi (Glomeromycota phylum), only found in the exterior soil, but not in the cave, were the genera Glomus, Claroideoglomus, and unidentified members of the family Glomeraceae, which are arbuscular mycorrhizal fungi (Pagano, 2016). Their obligate association with plants precludes their presence in the cave.
With low relative abundances there are a few interesting fungi in different locations along the cavity: Pseudogymnoascus pannorum and Leptobacillium leptobactrum.
Pseudogymnoascus pannorum is a psychrophilic, keratinolytic fungus (Garzoli et al., 2019), closely related to Pseudogymnoascus destructans, the causative agent of white-nose syndrome in bats. This is a soil borne fungus occurring worldwide and in caves. The fungus is common in European and North American caves and is adapted to grow between 4 and 15°C (Out et al., 2016; Vanderwolf et al., 2019; Sanchez-Moral et al., 2021).
The saprotrophic fungus Leptobacillium leptobactrum was detected in French and German caves (Bastian et al., 2009; Porca et al., 2011; Burow et al., 2019). In Spanish caves, the abundance of Leptobacillium leptobactrum and Leptobacillium symbioticum amounted 100% of the fungi isolated from the air in some halls (Dominguez-Moñino et al., 2021). This abundance was suggested to be related to their entomopathogenic activity.
Meyerozyma guilliermondii appeared exclusively in Sala Nevada. This is a cold-adapted ascomycete yeast often recorded in Arctic and Antarctic ecosystems (Sannino et al., 2021). Meyerozyma guilliermondii occurred in bat guano, in some cases with high isolation frequency (Larcher et al., 2003; Dimkić et al., 2020).
Spatial Distribution of Fungal Communities in Castañar Cave in 2020
In the 2020 sampling, the distribution of 12 fungal phyla in the soil outside the cave (Figure 4) is similar to that of forest soils. In fact, Kujawska et al. (2021) reported that in forest soils Ascomycota was the most abundant phylum, followed by Basidiomycota, Mucoromycota and Mortierellomycota. However, this diversity was greatly reduced inside the cave, with only two phyla (Ascomycota and Basidiomycota) in samples P1, P2, P5 and P6, and three phyla (Ascomycota, Basidiomycota and Mortierellomycota) in P4 and P7. This loss of diversity can be attributed, among other factors, to the lack of nutrients inside the cave, as opposite to the forest soil. The loss of diversity inside the cave increased in the inner part of the trail (P5-P6), corresponding to the most stable area with the least energy exchange with the exterior.
The uneven distribution of the phyla Ascomycota and Basidiomycota across the cave is remarkable. Most ascomycetes have been found in areas well connected to the entrance: Sala de Entrada (P1) and Galeria Principal (P2) or in areas with a high impact of tourist visits such as Sala Nevada (P4; Figure 1). Therefore, their presence in the cave is an indirect consequence of the entry of organic matter by three ways: cave animals, human visitors, and airborne spores from outside. The second part of the trail comprises a passage between Sala Nevada and Sala del Jardin (P5), Sala del Jardin (P6), and Sala Blanca (P7; Figure 1), where the relative abundances of basidiomycetes increased, especially in P5. The connection from Sala Nevada to Sala del Jardin (P5) is a very narrow passage that in some way separates both trail sides and this kind of isolation could be responsible of the different niches colonized by ascomycete and basidiomycete species.
It is worth mentioning the abundance of Mortierellomycota (30.80%) in Sala Nevada (P4), against Basidiomycota (1.01%), contrarily to those found in other halls where Basidiomycota ranged from 67.75 to 10.72% (Figure 4). Mortierellomycota was only found in Sala Nevada (P4), in addition to Sala Blanca (P7), where reached 2.42%. Most Mortierellomycota are saprobic soil-inhabiting fungi and many Mortierella alpina strains have been isolated from agricultural soils (Wagner et al., 2011). Other species of Mortierella were found in soil samples near the cave. Species of the genus Mortierella are common in soils and occur frequently in cave sediments (Nováková, 2009; Zhang et al., 2014; Man et al., 2018; Nováková et al., 2018).
Inside the cave, the fungal diversity was greater in Sala de Entrada (P1), an ecotone area very close to the entrance, where some roots and animals are frequently observed, as well as in P2, P4 and P7, while in the inner areas (P5 and P6) was low (Figure 5). Members of the phyla Basidiomycota and Ascomycota were found in the soil and Sala de Entrada sediment with high relative abundances. Glomeromycota were only found in the soil, as correspond to symbiotic arbuscular mycorrhizal fungi (Pagano, 2016). It was worthy of note the increase of Basidiomycota in all cave halls and galleries with respect to the exterior soil, except for Sala Nevada (P4).
It should be noted the high presence of endophytic fungus (Talaromyces, Porodiplodia vitis, Aureobasidium pullulans) and fungal species associated with roots and decaying plant material (Rhexocercosporidium panacis and Tritirachium dependens) in Sala de Entrada (Lu et al., 2014; Vanam et al., 2018; Crous et al., 2019). Therefore, their presence near the entrance was related with roots and the transport of organic matter from outside.
Regarding the ascomycetes, a few fungi were noticeable for their relative abundance: Candida parapsilosis, Cephalotrichum microsporum, an unidentified species of the genus Preussia and Neocosmospora solani were found at different locations along the cavity, while the unidentified species of the genera Diaporthe and Purpureocillium, as well as Trichophyton ajelloi only appeared in Sala Nevada (P4) and/or the connection between Sala Nevada and Sala del Jardin (P5).
Ecological Traits of Fungi
Castañar Cave is a highly radioactive site with peaks above 50 kBq/m3, but also a low-energy cave with oligotrophic conditions (before the vomit spillage) due to the high isolation level from the outdoor environment. These special conditions could affect fungal communities’ development.
Fungi are highly radioresistant when subjected to high doses of ionizing radiation under experimental or accidental conditions (Dadachova and Casadevall, 2008). Chernobyl (Dighton et al., 2008) and the Nevada Test Site (Durrell and Shields, 1960) affected by atmospheric and subterranean nuclear detonations are two reference sites where fungal studies have been carried out. Recently, Fukushima Dai-ichi Nuclear Power Plant was added to the list and it was shown that fungi accumulated high amounts of radiocesium (Fuma et al., 2017). In the literature there are a few papers on the microbial diversity in high 222Rn ecosystems (Anitori et al., 2002; Brugger et al., 2005; Weidler et al., 2007).
In the cave sediments the genera Preussia, Aspergillus, Acremonium, Mortierella and Penicillium were coincident with those found in Chernobyl as well as the species Pseudogymnoascus pannorum, Neocosmospora solani, Fusarium oxysporum, Penicillium citrinum, and Purpureocillium lilacinum (Zhdanova et al., 1994, 2000; Egorova et al., 2015). However, all these fungi can be considered as cave inhabitants, either in ionized caves or not, which indicates that these species are relatively tolerant to ionizing radiations.
A number of fungi have been studied in relation to the presence or activity in radioactive sites. Some of them have been found in Castañar Cave. Li et al. (2015) reported the isolation of the yeasts Meyerozyma (= Candida) guilliermondii and Rhodotorula calyptogenae from a radioactive waste repository. Shuryak et al. (2017) indicated that fungi were particularly resistant to ionizing radiation. These included Candida parapsilosis and Meyerozyma guilliermondii, in addition to a few Aspergillus, Acremonium and Chrysosporium species. The two first yeasts were found in the cave as well as different species of the three last fungal genera.
Zhdanova et al. (2000) detected fungal growth on the buildings of Chernobyl and isolated 37 fungi, from which Penicillium citrinum, Pseudogymnoascus pannorum, Neocosmospora solani, and Fusarium oxysporum were also found in the cave. All species, except Pseudogymnoascus pannorum, were isolated in locations severely contaminated. According to the authors, the annual dose received by these fungi is 105 times the natural background radiation and they noticed that about 80% of the fungi contained melanin.
Blachowicz et al. (2019) investigated the survival of 12 fungi isolated from Chernobyl to UV-C and simulated Mars conditions. Interestingly, among the fungi were represented Fusarium oxysporum and different species of Acremonium, Penicillium and Aspergillus.
Gessler et al. (2014) reported that Purpureocillium lilacinum strains isolated from Chernobyl areas had a melanin content 2–2.5 times higher than strains from other areas, concluding that radionuclide contamination changed the fungal communities by increasing the amount of melanized fungi, and Egorova et al. (2015) pointed out that this fungus, was used as a bioindicator of the high level of contamination with radionuclides in Chernobyl soils.
Traxler et al. (2021) proved that non-melanized fungi, non-adapted to high ionizing radiation, can survive, grow and spread in the Chernobyl Exclusion Zone soil, with a high level of ionizing radiation. The authors inoculated a model basidiomycete Schizophyllum commune into the soil and confirmed that the fungus was present 1 year after inoculation and could cross 1 m distance within 6 months. The test showed that unadapted fungi can thrive in highly contaminated soils where radiation and heavy metals were present.
In light of our data, a high number of non-melanized fungi thrived in the cave sediments but melanized fungi were scarce. This can be explained because cave fungi do not need melanins to protect from UV radiation.
The ecological traits, as well the niches of the most abundant fungal species in Castañar Cave (from P2 to P7) are summarized in Supplementary Tables S3 and S4. Regarding the potential danger to human health, a few species were human opportunistic fungal pathogens: Candida parapsilosis, Meyerozyma guilliermondii, Pseudogymnoascus pannorum, Leptobacillium leptobactrum, Malassezia globosa. Almost all the fungi in this cave were saprophytes and endophytes and were isolated from caves and soils. As opposed to that observed in other show caves (Dominguez-Moñino et al., 2021), Castañar Cave presented a low occurrence of entomopathogenic fungi: Leptobacillium leptobactrum and Purpureocillium, both in areas well connected to the outside.
Other different ecological traits were noticed with the identification of psychrophilic (Candida parapsilosis, Malassezia globosa, Pseudogymnoascus pannorum, Meyerozyma guilliermondii, Buckleyzyma, Penicillium sp., Mortierella, Neocosmospora solani, Fusarium oxysporum, Preussia sp.), keratinolytic (Trichophyton ajelloi, Pseudogymnoascus pannorum, Chrysosporium pseudomerdarium, Penicillium spp., Neocosmospora solani, Candida parapsilosis, Penicillium citrinum, Talaromyces), or coprophilous and/or guano-inhabitant taxa (Mortierella alpina, Preussia sp., Penicillium citrinum, Chrysosporium pseudomerdarium, Meyerozyma guilliermondii, Candida parapsilosis, Talaromyces spp., Cephalotrichum microsporum).
In general, most fungi retrieved are typical soil-borne fungi and widespread in nature (e.g., Purpureocillium lilacinum, Penicillium decumbens, Neocosmospora solani, Chaetomium globosum, Pochonia chlamydosporia, Cephalotrichum stemonitis, Cephalotrichum microsporum, Penicillium citrinum, Cladosporium cladosporioides, Cladosporium sphaerospermum, Aspergillus ustus, etc.; Domsch et al., 2007).
Conclusion
The mycobiota of Castañar Cave is defined by the input of organic matter from anthropogenic and animal sources (including dung). It is hypothesized that ecologically distinct niches were created in the cave by the input of diverse types of organic matter, under different environmental conditions and mineral substrata. These niches may account for certain fungal species being present or absent along the cave. The zones well connected to the exterior and Sala Nevada with high impact of tourist visits host copiotrophic fungal species while those thriving in the inner zones occurred under relatively oligotrophic conditions. Under these limited conditions, we observed occasional fungal inhabitants because of the entry of foreign organic matter during visits. The loss of diversity inside the cave increased in the inner part corresponding to the most stable area with the least energy exchange with the exterior.
Regarding persistence, the occurrence of Neocosmospora solani in all samplings from 2008 till 2020 is noteworthy since it has been widely reported in relation to cave outbreaks. In addition, this fungus is usually a very abundant soil inhabitant and is able of taking advantage of any disturbance to over develop, as it made during the vomit spillage. An additional attention merits the abundance of Cephalotrichum microsporum along the cave. This and other abundant taxa should be controlled.
Fungi previously reported in highly radioactive environments were also found in Castañar Cave, but the effect of high 222Rn on these fungi is not conclusive taking into account that the diversity is similar to that found in other caves with relatively low 222Rn concentrations.
Data Availability Statement
The datasets presented in this study can be found in online repositories. The name of the repository and accession number can be found in the article.
Author Contributions
TM-P, AN, and VJ: samplings and microbial analyses. AF-C, SS-M, and SC: environmental analyses. TM-P and CS-J: writing of the manuscript with support from SS-M and AF-C. SS-M and CS-J: research coordination and funding. All authors have contributed to the scientific discussion of the data and agreed to the submitted version of the manuscript.
Funding
This research was supported by the Spanish Ministry of Science and Innovation through project PID2019-110603RB-I00 and the collaboration of PID2020-114978GB-I00 project, MCIN/AEI/FEDER, UE/10.13039/501100011033.
Conflict of Interest
The authors declare that the research was conducted in the absence of any commercial or financial relationships that could be construed as a potential conflict of interest.
Publisher’s Note
All claims expressed in this article are solely those of the authors and do not necessarily represent those of their affiliated organizations, or those of the publisher, the editors and the reviewers. Any product that may be evaluated in this article, or claim that may be made by its manufacturer, is not guaranteed or endorsed by the publisher.
Acknowledgments
The authors acknowledge to CSIC Open Access Publication Support Initiative through its Unit of Information Resources for Research (URICI), and CSIC Interdisciplinary Thematic Platform Open Heritage: Research and Society (PTI-PAIS) for the professional support.
Supplementary Material
The Supplementary Material for this article can be found online at: https://www.frontiersin.org/articles/10.3389/fmicb. 2022.869661/full#supplementary-material
References
Abdo, H., Catacchio, C. R., Ventura, M., D’Addabbo, P., Alexandre, H., Guilloux-Bénatier, M., et al. (2020). The establishment of a fungal consortium in a new winery. Sci. Rep. 10:7962. doi: 10.1038/s41598-020-64819-2
Alonso-Zarza, A. M., and Martin-Perez, A. (2008). Dolomite in caves: recent dolomite formation in oxic, non-sulfate environments. Castañar Cave, Spain. Sediment. Geol. 205, 160–164. doi: 10.1016/j.sedgeo.2008.02.006
Alonso-Zarza, A. M., Martín-Pérez, A., Martín-García, R., Gil-Peña, I., Meléndez, A., Martínez-Flores, E., et al. (2011). Structural and host rock controls on the distribution, morphology and mineralogy of speleothems in the Castañar Cave (Spain). Geol. Mag. 148, 211–225. doi: 10.1017/S0016756810000506
Anitori, R. P., Trott, C., Saul, D. J., Bergquist, P. L., and Walter, H. R. (2002). A culture-independent survey of the bacterial community in a radon hot spring. Astrobiology 2, 255–270. doi: 10.1089/153110702762027844
Bastian, F., Alabouvette, C., and Saiz-Jimenez, C. (2009). The impact of arthropods on fungal community structure in Lascaux Cave. J. Appl. Microbiol. 106, 1456–1462. doi: 10.1111/j.1365-2672.2008.04121.x
Blachowicz, A., Chiang, A. J., Elsaesser, A., Kalkum, M., Ehrenfreund, P., Stajich, J. E., et al. (2019). Proteomic and metabolomic characteristics of extremophilic fungi under simulated mars conditions. Front. Microbiol. 10:1013. doi: 10.3389/fmicb.2019.01013
Bohacz, J. (2017). Biodegradation of feather waste keratin by a keratinolytic soil fungus of the genus Chrysosporium and statistical optimization of feather mass loss. World J. Microbiol. Biotechnol. 33:13. doi: 10.1007/s11274-016-2177-2
Bolyen, E., Rideout, J. R., Dillon, M. R., Bokulich, N. A., Abnet, C., Al-Ghalith, G. A., et al. (2019). Reproducible, interactive, scalable, and extensible microbiome data science using QUIIME 2. Nat. Biotechnol. 37, 852–857. doi: 10.1038/s41587-019-0209-9
Brugger, J., Long, N., McPhail, D. C., and Plimer, I. (2005). An active amagmatic hydrothermal system: the Paralana hot springs, northern Flinders ranges, South Australia. Chem. Geol. 222, 35–64. doi: 10.1016/j.chemgeo.2005.06.007
Burow, K., Grawunder, A., Harpke, M., Pietschmann, S., Ehrhardt, R., Wagner, L., et al. (2019). Microbiomes in an acidic rock–water cave system. FEMS Microbiol. Lett. 366:fnz167. doi: 10.1093/femsle/fnz167
Callahan, B. J., Mcmurdie, P. J., Rosen, M. J., Han, A. W., Johnson, A. J., and Holmes, S. P. (2016). DADA2 paper supporting information: high-resolution sample inference from amplicon data. Nat. Methods 13, 581–583. doi: 10.1038/nmeth.3869
Castro, M. L., Barreiro, F., and Martínez, J. J. (2011). Omphalotus olearius (DC.: Fr.) Singer: especie alóctona da micobiota de Galicia (España)? Mykes 14, 7–11.
Connell, L. B., Rodriguez, R. R., Redman, R. S., and Dalluge, J. J. (2014). “Cold-adapted yeasts in Antarctic deserts,” in Cold-Adapted Yeasts. eds. P. Buzzini and R. Margesin (Heidelberg: Springer), 75–98.
Connell, L., and Staudigel, H. (2013). Fungal diversity in a dark oligotrophic volcanic ecosystem (DOVE) on mount Erebus, Antarctica. Biology 2, 798–809. doi: 10.3390/biology2020798
Crous, P. W., Schumacher, R. K., Akulov, A., Thangavel, R., Hernández-Restrepo, M., Carnegie, A. J., et al. (2019). New and interesting fungi. 2. Fungal Syst. Evol. 3, 57–134. doi: 10.3114/fuse.2019.03.06
Cunha, A. O. B., Bezerra, J. D. P., Oliveira, T. G. L., Barbier, E., Bernard, E., Machado, A. R., et al. (2020). Living in the dark: bat caves as hotspots of fungal diversity. PLoS One 15:e0243494. doi: 10.1371/journal.pone.0243494
Dadachova, E., and Casadevall, A. (2008). Ionizing radiation: how fungi cope, adapt, and exploit with the help of melanin. Curr. Opin. Microbiol. 11, 525–531. doi: 10.1016/j.mib.2008.09.013
Degawa, Y., and Gams, W. (2004). A new species of Mortierella, and an associated sporangiiferous mycoparasite in a new genus, Nothadelphia. Stud. Mycol. 50, 567–572.
Dighton, J., Tugay, T., and Zhdanova, N. (2008). Fungi and ionizing radiation from radionuclides. FEMS Microbiol. Lett. 281, 109–120. doi: 10.1111/j.1574-6968.2008.01076.x
Dimkić, I., Stanković, S., Kabić, J., Stupar, M., Nenadić, M., Ljaljević-Grbić,, et al. (2020). Bat guano-dwelling microbes and antimicrobial properties of the pygidial gland secretion of a troglophilic ground beetle against them. Appl. Microbiol. Biotechnol. 104, 4109–4126. doi: 10.1007/s00253-020-10498-y
Dominguez-Moñino, I., Jurado, V., Rogerio-Candelera, M. A., Hermosin, B., and Saiz-Jimenez, C. (2021). Airborne fungi in show caves from southern Spain. Appl. Sci. 11:5027. doi: 10.3390/app11115027
Domsch, K. H., Gams, W, and Anderson, T.-H. (2007). Compendium of Soil Fungi. 2nd Edn. Eching: IHW-Verlag.
Dupont, J., Jacquet, C., Dennetiere, B., Lacoste, S., Bousta, F., Orial, G., et al. (2007). Invasion of the French Paleolithic painted cave of Lascaux by members of the Fusarium solani species complex. Mycologia 99, 526–533. doi: 10.1080/15572536.2007.11832546
Durrell, L. W., and Shields, L. M. (1960). Fungi isolated in culture from soils of the Nevada test site. Mycologia 52, 636–641. doi: 10.2307/3756096
Egorova, A. S., Gessler, N. N., Ryasanova, L. P., Kulakovskaya, T. V., and Belozerskaya, T. A. (2015). Stress resistance mechanisms in the indicator fungi from highly radioactive Chernobyl zone sites. Microbiology 84, 152–158. doi: 10.1134/S0026261715020034
Eslyn, W. E., and Lombard, F. F. (1983). Decay in mine timbers. Part II. Basidiomycetes associated with decay of coal mine timbers. Forest Prod. J. 33, 19–23.
Fernandez-Cortes, A., Sanchez-Moral, S., Cuezva, S., Benavente, D., and Abella, R. (2011). Characterization of tracer gases fluctuations on a “low energy” cave (Castañar de Ibor, Spain) using techniques of entropy of curves. Int. J. Climatol. 31, 127–143. doi: 10.1002/joc.2057
Fernandez-Cortes, A., Sanchez-Moral, S., Cuezva, S., Cañaveras, J. C., and Abella, R. (2009). Annual and transient signatures of gas exchange and transport in the Castañar de Ibor cave (Spain). Int. J. Speleol. 38, 153–162. doi: 10.5038/1827-806X.38.2.6
Fuma, S., Ihara, S., Takahashi, H., Inaba, O., Sato, Y., Kubota, Y., et al. (2017). Radiocaesium contamination and dose rate estimation of terrestrial and freshwater wildlife in the exclusion zone of the Fukushima Dai-ichi nuclear power plant accident. J. Environ. Radioac. 171, 176–188. doi: 10.1016/j.jenvrad.2017.02.013
Garcia-Guinea, J., Fernandez-Cortes, A., Alvarez-Gallego, M., Garcia-Antón, E., Casas-Ruiz, M., Blázquez-Pérez, D., et al. (2013). Leaching of uranyl–silica complexes from the host metapelite rock favoring high radon activity of subsoil air: case of Castañar cave (Spain). J. Radioanal. Nucl. Chem. 298, 1567–1585. doi: 10.1007/s10967-013-2587-7
Garzoli, L., Riccucci, M., Patriarca, E., Debernardi, P., Boggero, A., Pecoraro, L., et al. (2019). First isolation of Pseudogymnoascus destructans, the fungal causative agent of white-nose disease, in bats from Italy. Mycopathologia 184, 637–644. doi: 10.1007/s11046-019-00371-6
Gessler, N. N., Egorova, A. S., and Belozerskaya, T. A. (2014). Melanin pigments of fungi under extreme environmental conditions (review). Appl. Biochem. Microbiol. 50, 105–113. doi: 10.1134/S0003683814020094
Giurgiu, A., and Tamas, T. (2013). Mineralogical data on bat guano deposits from three Romanian caves. Studia UBB Geol. 58, 13–18. doi: 10.5038/1937-8602.58.2.2
Gomes, R. R., Glienke, C., Videira, S. I. R., Lombard, L., Groenewald, J. Z., and Crous, P. W. (2013). Diaporthe: a genus of endophytic, saprobic and plant pathogenic fungi. Persoonia 31, 1–41. doi: 10.3767/003158513X666844
Gonzalez-Menendez, V., Martin, J., Siles, J. A., Gonzalez-Tejero, M. R., Reyes, F., Platas, G., et al. (2017). Biodiversity and chemotaxonomy of Preussia isolates from the Iberian Peninsula. Mycol. Prog. 16, 713–728. doi: 10.1007/s11557-017-1305-1
Held, B. W., Salomon, C. E., and Blanchette, R. A. (2020). Diverse subterranean fungi of an underground iron ore mine. PLoS One 15:e0234208. doi: 10.1371/journal.pone.0234208
Heredia, G., Arias-Mota, R. M., Mena-Portales, J., and Castañeda-Ruiz, R. F. (2018). Saprophytic synnematous microfungi. New records and known species for Mexico. Rev. Mex. Biodivers. 89, 604–618. doi: 10.22201/ib.20078706e.2018.3.2352
Hill, C. A., and Forti, P. (1997). Cave Minerals of the World. 2nd Edn. Huntsville: National Speleological Society.
Holz, P. H., Lumsden, L. F., Marenda, M. S., Browning, G. F., and Hufschmid, J. (2018). Two subspecies of bent-winged bats (Miniopterus orianae bassanii and oceanensis) in southern Australia have diverse fungal skin flora but not Pseudogymnoascus destructans. PLoS One 13:e0204282. doi: 10.1371/journal.pone.0204282
Jiang, J.-R., Cai, L., and Liu, F. (2017). Oligotrophic fungi from a carbonate cave, with three new species of Cephalotrichum. Mycology 8, 164–177. doi: 10.1080/21501203.2017.1366370
Jurado, V., Del Rosal, Y., Liñan, C., Martin-Pozas, T., Gonzalez-Pimentel, J. L., and Saiz-Jimenez, C. (2021). Diversity and seasonal dynamics of airborne fungi in Nerja Cave, Spain. Appl. Sci. 11:6236. doi: 10.3390/app11136236
Jurado, V., Porca, E., Cuezva, S., Fernandez-Cortes, A., Sanchez-Moral, S., and Saiz-Jimenez, C. (2010). Fungal outbreak in a show cave. Sci. Total Environ. 408, 3632–3638. doi: 10.1016/j.scitotenv.2010.04.057
Karunarathna, S. C., Dong, Y., Karasaki, S., Tibpromma, S., Hyde, K. D., Lumyong, S., et al. (2020). Discovery of novel fungal species and pathogens on bat carcasses in a cave in Yunnan Province, China. Emerg. Microbes Infect. 9, 1554–1566. doi: 10.1080/22221751.2020.1785333
Khan, S. R. (1998). “Phosphate urolithiasis, rat,” in Urinary System. Monographs on Pathology of Laboratory Animals. eds. T. C. Jones, G. C. Hard, and U. Mohr (Berlin: Springer), 451–456.
Korhonen, A., Seelan, J. S. S., and Miettinen, O. (2018). Cryptic species diversity in polypores: the Skeletocutis nivea species complex. MycoKeys 36, 45–82. doi: 10.3897/mycokeys.36.27002
Kujawska, B., Rudawska, M., Wilgan, R., and Leski, T. (2021). Similarity and differences among soil fungal assemblages in managed forests and formerly managed forest reserves. Forests 12:353. doi: 10.3390/f12030353
Larcher, G., Bouchara, J. P., Pailley, P., Montfort, D., Behuin, H., De Biève, C., et al. (2003). Fungal biota associated with bats in Western France. J. Med. Mycol. 13, 29–34.
Lario, J., Sanchez-Moral, S., Cuezva, S., Taborda, M., and Soler, V. (2006). High 222Rn levels in a show cave (Castañar de Ibor, Spain): proposal and application on management measures to minimize the effects on guides and visitors. Atmos. Environ. 40, 7395–7400. doi: 10.1016/j.atmosenv.2006.06.046
Li, C.-C., Chung, H.-P., Wen, H.-W., Chang, C.-Y., Wang, Y.-T., and Chou, F.-I. (2015). The radiation resistance and cobalt biosorption activity of yeast strains isolated from the Lanyu low-level radioactive waste repository in Taiwan. J. Environ. Radioact. 146, 80–87. doi: 10.1016/j.jenvrad.2015.04.010
Li, A.-H., Yuan, F.-X., Groenewald, M., Bensch, K., Yurkov, A. M., Li, K., et al. (2020). Diversity and phylogeny of basidiomycetous yeasts from plant leaves and soil: proposal of two new orders, three new families, eight new genera and one hundred and seven new species. Stud. Mycol. 96, 17–140. doi: 10.1016/j.simyco.2020.01.002
Lu, X. H., Chen, A. J., Zhang, X. S., Jiao, X. L., and Gao, W. W. (2014). First report of Rhexocercosporidium panacis causing rusty root of Panax ginseng in northeastern China. Plant Dis. 98:1580. doi: 10.1094/PDIS-01-14-0082-PDN
Ludwig, L., Muraoka, J. Y., Bonacorsi, C., and Donofrio, F. C. (2023). Diversity of fungi obtained from bats captured in urban forest fragments in Sinop, Mato Grosso, Brazil. Braz. J. Biol. 83:e247993. doi: 10.1590/1519-6984.247993
Man, B., Wang, H., Yun, Y., Xiang, X., Wang, R., Duan, Y., et al. (2018). Diversity of fungal communities in Heshang Cave of Central China revealed by mycobiome-sequencing. Front. Microbiol. 9:1400. doi: 10.3389/fmicb.2018.01400
Martin-Sanchez, P. M., Jurado, V., Porca, E., Bastian, F., Lacanette, D., Alabouvette, C., et al. (2014). Aerobiology of Lascaux Cave (France). Int. J. Speleol. 43, 295–303. doi: 10.5038/1827-806X.43.3.6
Mašínová, T., Bahnmann, B. D., Větrovský, T., Tomšovský, M., Merunková, K., and Baldrian, P. (2017). Drivers of yeast community composition in the litter and soil of a temperate forest. FEMS Microbiol. Ecol. 93:fiw223. doi: 10.1093/femsec/fiw223
McMurdie, P. J., and Holmes, S. (2013). Phyloseq: an R package for reproducible interactive analysis and graphics of microbiome census data. PLoS One 8:e61217. doi: 10.1371/journal.pone.0061217
Nagai, K., Suzuki, K., and Okada, G. (1998). Studies on the distribution of alkalophilic and alkali-tolerant soil fungi II: fungal flora in two limestone caves in Japan. Mycoscience 39, 293–298. doi: 10.1007/BF02464011
Nguyen, H. D., Nickerson, N. L., and Seifert, K. A. (2013). Basidioascus and Geminibasidium: a new lineage of heat-resistant and xerotolerant basidiomycetes. Mycologia 105, 1231–1250. doi: 10.3852/12-351
Nováková, A. (2009). Microscopic fungi isolated from the Domica cave system (Slovak karst National Park, Slovakia). A review. Int. J. Speleol. 38, 71–82. doi: 10.5038/1827-806X.38.1.8
Nováková, A. (2021). Výskyt hub v jeskyních a jiných podzemních prostorách Slovenské republiky (Fungal occurrence in caves and other underground spaces in the Slovak Republic). Acta Carsologica Slovaca 59, 5–58.
Nováková, A., Hubka, V., Valinová, S., Kolařík, M., and Hillebrand-Voiculescu, A. M. (2018). Cultivable microscopic fungi from an underground chemosynthesis-based ecosystem: a preliminary study. Folia Microbiol. 63, 43–55. doi: 10.1007/s12223-017-0527-6
Out, B., Boyle, S., and Cheeptham, N. (2016). Identification of fungi from soil in the Nakimu caves of glacier National Park. UJEMI+ 2, 26–32.
Péter, G., Takashima, M., and Cadez, N. (2017). “Yeast habitats: different but global,” in Yeasts in Natural Ecosystems: Ecology. eds. P. Buzzini, M.-A. Lachance, and A. Yurkov (Cham: Springer), 39–71.
Popkova, A. V., and Mazina, S. E. (2019). Microbiota of hypogean habitats in Otap Head Cave. J. Environ. Res. Eng. Manag. 75, 71–82. doi: 10.5755/j01.erem.75.3.21106
Porca, E., Jurado, V., Martin-Sanchez, P. M., Hermosin, B., Bastian, F., Alabouvette, C., et al. (2011). Aerobiology: an ecological indicator for early detection and control of fungal outbreaks in caves. Ecol. Indic. 11, 1594–1598. doi: 10.1016/j.ecolind.2011.04.003
Ren, H., Wang, H., Yu, Z., Zhang, S., Qi, X., Sun, L., et al. (2021). Effect of two kinds of fertilizers on growth and rhizosphere soil properties of bayberry with decline disease. Plan. Theory 10:2386. doi: 10.3390/plants10112386
Romsdahl, J., Blachowicz, A., Chiang, A. J., Singh, N., Stajich, J. E., Kalkum, M., et al. (2018). Characterization of Aspergillus niger Isolated from the International Space Station. mSystems 3, e00112–e00118. doi: 10.1128/mSystems.00112-18
Sanchez-Moral, S., Jurado, V., Fernandez-Cortes, A., Cuezva, S., Martin-Pozas, T., Gonzalez-Pimentel, J. L., et al. (2021). Environment-driven control of fungi in subterranean ecosystems: the case of La Garma cave (northern Spain). Int. Microbiol. 24, 573–591. doi: 10.1007/s10123-021-00193-x
Sannino, C., Borruso, L., Mezzasoma, A., Battistel, D., Ponti, S., Turchetti, B., et al. (2021). Abiotic factors affecting the bacterial and fungal diversity of permafrost in a rock glacier in the Stelvio Pass (Italian Central Alps). Appl. Soil Ecol. 166:104079. doi: 10.1016/j.apsoil.2021.104079
Saxena, P., Kumar, A., and Shrivastava, J. N. (2004). Diversity of keratinophilic mycoflora in the soil of Agra (India). Folia Microbiol. 49, 430–434. doi: 10.1007/BF02931605
Shuryak, I., Matrosova, V. Y., Gaidamakova, E. K., Tkavc, R., Grichenko, O., Klimenkova, P., et al. (2017). Microbial cells can cooperate to resist high- level chronic ionizing radiation. PLoS One 12:e0189261. doi: 10.1371/journal.pone.0189261
Siasou, E., Johnson, D., and Willey, N. J. (2017). An extended dose–response model for microbial responses to ionizing radiation. Front. Environ. Sci. 5:6. doi: 10.3389/fenvs.2017.00006
Somlai, J., Hakl, J., Kávási, N., Szeiler, G., Szabó, P., and Kovács, T. (2011). Annual average radon concentration in the show caves of Hungary. J. Radioanal. Nucl. Chem. 287, 427–433. doi: 10.1007/s10967-010-0841-9
Traxler, L., Wollenberg, A., Steinhauser, G., Chyzhevskyi, I., Dubchak, S., Großmann, S., et al. (2021). Survival of the basidiomycete Schizophyllum commune in soil under hostile environmental conditions in the Chernobyl exclusion zone. J. Hazard. Mater. 403:124002. doi: 10.1016/j.jhazmat.2020.124002
Turenne, C. Y., Sanche, S. E., Hoban, D. J., Karlowsky, J. A., and Kabani, A. M. (1999). Rapid identification of fungi by using the ITS2 genetic region and an automated fluorescent capillary electrophoresis system. J. Clin. Microbiol. 37, 1846–1851. doi: 10.1128/JCM.37.6.1846-1851.1999
Ulloa, M., Lappe, P., Aguilar, S., Park, H., Pérez-Mejía, A., Toriello, C., et al. (2006). Contribution to the study of the mycobiota present in the natural habitats of Histoplasma capsulatum: an integrative study in Guerrero, Mexico. Rev. Mex. Biodivers. 77, 153–168.
Urbaniak, C., van Dam, P., Zaborin, A., Zaborina, O., Gilbert, J. A., Torok, T., et al. (2019). Genomic characterization and virulence potential of two Fusarium oxysporum isolates cultured from the International Space Station. mSystems 4, e00345–e00318. doi: 10.1128/mSystems.00345-18
Van Houdt, R., Mijnendonckx, K., and Leys, N. (2012). Microbial contamination monitoring and control during human space missions. Planet. Space Sci. 60, 115–120. doi: 10.1016/j.pss.2011.09.001
Vanam, H. P., Rao, P. N., Mohanran, K., Yegneswaran, P. P., and Rudramurthy, S. P. M. (2018). Distal lateral subungual onychomycosis owing to Tritirachium oryzae: A bystander or invader? Mycopathologia 183, 459–463. doi: 10.1007/s11046-017-0226-5
Vanderwolf, K. J., Malloch, D., and McAlpine, D. F. (2019). No change detected in culturable fungal assemblages on cave walls in eastern Canada with the introduction of Pseudogymnoascus destructans. Diversity 11:222. doi: 10.3390/d11120222
Wagner, L., Stielow, B., Hoffmann, K., Petkovits, T., Papp, T., Vágvölgyi, C., et al. (2011). Molecular characterization of airborne fungi in caves of the Mogao grottoes, Dunhuang, China. Int. Biodeter. Biodegr. 65, 726–731. doi: 10.1016/j.ibiod.2011.04.006
Wang, Q.-M., Yurkov, A. M., Göker, M., Lumbsch, H. T., Leavitt, S. D., Groenewald, M., et al. (2016). Phylogenetic classification of yeasts and related taxa within Pucciniomycotina. Stud. Mycol. 81, 149–189. doi: 10.1016/j.simyco.2015.12.002
Wasti, I. G., Fui, F. S., Zhi, T. Q., Mun, C. W., Kassim, M. H. S., Dawood, M. M., et al. (2020). Fungi from dead arthropods and bats of Gomantong cave, northern Borneo, Sabah (Malaysia). J. Cave Karst Stud. 82, 261–275. doi: 10.4311/2019MB0146
Weidler, G. W., Dornmayr-Pfaffenhuemer, M., Gerbl, F. W., Heinen, W., and Stan-Lotter, H. (2007). Communities of archaea and bacteria in a subsurface radioactive thermal spring in the Austrian Central Alps, and evidence of ammonia-oxidizing Crenarchaeota. Appl. Environ. Microbiol. 73, 259–270. doi: 10.1128/AEM.01570-06
White, T. J., Bruns, T., Lee, S., and Taylor, J. (1990). “Amplification and direct sequencing of fungal ribosomal RNA genes for phylogenetics,” in PCR Protocols: A Guide to Methods and Applications. eds. M. A. Innis, D. H. Gelfand, J. J. Sninsky, and T. J. White (London: Academic Press), 315–322.
Zhang, Z. F., Liu, F., Zhou, X., Liu, X. Z., Liu, S. J., and Cai, L. (2017). Culturable mycobiota from karst caves in China, with descriptions of 20 new species. Persoonia 39, 1–31. doi: 10.3767/persoonia.2017.39.01
Zhang, T., Victor, T. R., Rajkumar, S. S., Li, X., Okoniewski, J. C., Hicks, A. C., et al. (2014). Mycobiome of the bat white nose syndrome affected caves and mines reveals diversity of fungi and local adaptation by the fungal pathogen Pseudogymnoascus (Geomyces) destructans. PLoS One 9:e108714. doi: 10.1371/journal.pone.0108714
Zhdanova, N. N., Vasilevskaya, A. I., Lashko, T. N., Gavrilyuk, V. I., and Dighton, J. (1994). Changes in micromycetes communities in soil in response to pollution by long-lived radionuclides emitted in the Chernobyl accident. Mycol. Res. 98, 789–795. doi: 10.1016/S0953-7562(09)81057-5
Zhdanova, N. N., Zakharchenko, V. A., Vember, V. V., and Nakonechnaya, L. T. (2000). Fungi from Chernobyl: mycobiota of the inner regions of the containment structures of the damaged nuclear reactor. Mycol. Res. 104, 1421–1426. doi: 10.1017/S0953756200002756
Keywords: fungal outbreak, Castañar Cave, radon, ionizing radiation, Ascomycota, Basidiomycota
Citation: Martin-Pozas T, Nováková A, Jurado V, Fernandez-Cortes A, Cuezva S, Saiz-Jimenez C and Sanchez-Moral S (2022) Diversity of Microfungi in a High Radon Cave Ecosystem. Front. Microbiol. 13:869661. doi: 10.3389/fmicb.2022.869661
Edited by:
Saskia Bindschedler, Université de Neuchâtel, SwitzerlandReviewed by:
Nihal Doğruöz Güngör, Istanbul University, TurkeyJohann Leplat, Laboratoire de Recherche des Monuments Historiques, France
Copyright © 2022 Martin-Pozas, Nováková, Jurado, Fernandez-Cortes, Cuezva, Saiz-Jimenez and Sanchez-Moral. This is an open-access article distributed under the terms of the Creative Commons Attribution License (CC BY). The use, distribution or reproduction in other forums is permitted, provided the original author(s) and the copyright owner(s) are credited and that the original publication in this journal is cited, in accordance with accepted academic practice. No use, distribution or reproduction is permitted which does not comply with these terms.
*Correspondence: Cesareo Saiz-Jimenez, saiz@irnase.csic.es