- 1Department of Chemical Biology and Genetics, Centre of the Region Haná for Biotechnological and Agricultural Research, Faculty of Science, Palacký University, Olomouc, Czechia
- 2Laboratory of Growth Regulators, Palacký University and Institute of Experimental Botany, Czech Academy of Sciences, Olomouc, Czechia
Drought and salinity reduce seed germination, seedling emergence, and early seedling establishment, affect plant metabolism, and hence, reduce crop yield. Development of technologies that can increase plant tolerance of these challenging growth conditions is a major current interest among plant scientists and breeders. Seed priming has become established as one of the practical approaches that can alleviate the negative impact of many environmental stresses and improve the germination and overall performance of crops. Hormopriming using different plant growth regulators has been widely demonstrated as effective, but information about using cytokinins (CKs) as priming agents is limited to only a few studies using kinetin or 6-benzylaminopurine (BAP). Moreover, the mode of action of these compounds in improving seed and plant fitness through priming has not yet been studied. For many years, BAP has been one of the CKs most commonly applied exogenously to plants to delay senescence and reduce the impact of stress. However, rapid endogenous N9-glucosylation of BAP can result in negative effects. This can be suppressed by hydroxylation of the benzyl ring or by appropriate N9 purine substitution. Replacement of the 2′ or 3′ hydroxyl groups of a nucleoside with a fluorine atom has shown promising results in drug research and biochemistry as a means of enhancing biological activity and increasing chemical or metabolic stability. Here, we show that the application of this chemical modification in four new N9-substituted CK derivatives with a fluorinated carbohydrate moiety improved the antisenescence properties of CKs. Besides, detailed phenotypical analysis of the growth and development of Arabidopsis plants primed with the new CK analogs over a broad concentration range and under various environmental conditions revealed that they improve growth regulation and antistress activity. Seed priming with, for example, 6-(3-hydroxybenzylamino)-2′-deoxy-2′-fluoro-9-(β)-D-arabinofuranosylpurine promoted plant growth under control conditions and alleviated the negative effects of the salt and osmotic stress. The mode of action of this hormopriming and its effect on plant metabolism were further analyzed through quantification of the endogenous levels of phytohormones such as CKs, auxins and abscisic acid, and the results are discussed.
Introduction
Global climate change is increasing the severity of drought and soil salinity, with deleterious effects on already-stressed agricultural ecosystems. Moreover, predictions for the future indicate that the areas affected by these two types of stress are going to expand and as a consequence the productivity of many plant species will be reduced (Savvides et al., 2016; Uddin et al., 2016; Pavlů et al., 2018). The development of biotechnological approaches that increase plant tolerance and assure the maintenance of yield under these challenging growth conditions is therefore one of the main aims of plant scientists and breeders.
One of the technologies that attracts a high level of interest nowadays is “seed priming” (Savvides et al., 2016). Seed priming is an effective pre-sowing technology in which seeds are treated with small doses of certain agents just prior to germination. Unlike un-primed seeds, primed seeds are able to respond to very low levels of specific stimuli, which helps plants to prepare their metabolism for better defense responses to stress factors (Conrath, 2011; Paparella et al., 2015). Thus, priming can improve seed performance, ensure higher uniformity among the seeds, result in faster and better synchronized germination, and enhance plant growth (Gamir et al., 2014; Ibrahim, 2016; Lutts et al., 2016). Several methods of seed priming, including hydropriming, osmopriming, hormopriming, biopriming, and chemical priming, have been developed (Jisha et al., 2013; Paparella et al., 2015). Hormopriming consists in the exogenous application of plant growth regulators or phytohormones that can stimulate seed imbibition and modify seed metabolism. The plant growth regulators most often used in this way are abscisic acid (ABA), gibberellins, cytokinins (CKs), auxins, ethylene, and polyamines (reviewed by De Diego and Spíchal, 2020).
In plants, CKs are involved in many biological processes: regulating sink/source relationships (Roitsch and Ehneß, 2000; Werner et al., 2008), nutrient uptake (Sakakibara, 2006; Criado et al., 2009), leaf senescence (Jordi et al., 2000; Marchetti et al., 2018), and responses to abiotic stress (Bielach et al., 2017). Since the discovery of the first CK, kinetin, by Skoog, Miller, and associates in 1955 (Miller et al., 1955), the number of chemicals fitting the definition of CK has grown to include a large array of natural and synthetic compounds, among which are adenine and phenylurea derivatives (Mok and Mok, 2001). Depending on their chemical structure, natural CKs are adenine derivatives with an isoprenoid or aromatic N6-side chain (Mok and Mok, 2001). CKs are present in plants in the forms of free bases, glucosides, nucleosides and nucleotides, at very low concentrations [pmol g–1 fresh weight (FW)] (Strnad, 1997). The precursor nucleotides, namely N9-riboside-5′- mono-, di-, and tri-phosphates, are endogenously synthesized de novo and converted to active free bases. The bases can be subsequently conjugated with glucose at positions N3, N7, and N9 of the purine ring and at the hydroxyl group of the side chain, which can be also conjugated with xylose (Frébort et al., 2011). Addition of sugar moieties to the N9 position of the purine ring can also form N9-riboside-glucoside (George et al., 2008). While the O-glycosylated forms can be converted back into active CKs, N-glycosylation occurs primarily at positions N7 or N9 of the purine ring, and is thought to be irreversible (Brzobohatý et al., 1993), except in the case of the tZ forms (Hošek et al., 2020). Furthermore, it has been demonstrated that some of these conjugates may have significant CK activity, especially in the case of N9-riboside analogs (Doležal et al., 2007).
The aromatic CK benzylaminopurine (BAP) is considered the most effective and the cheapest CK, which has led to its widespread use in biotechnology. However, many disadvantages associated with its applications have been reported (Werbrouck et al., 1995; Bairu et al., 2009). Negative effects can be caused by natural N9-glucosylation of the applied purine based CK, leading to extensive accumulation of non-active CK glucosides (Werbrouck et al., 1995). Moreover, N9-glucosides can activate ethylene production and the ethylene signaling pathway causing inhibition of root elongation (Podlešáková et al., 2012). One way of avoiding the negative effects of N9-glucosylation is to suppress it by appropriate N9 purine substitution in BAP or by hydroxylation of its benzyl ring (Plíhal et al., 2013).
Fluorination has a long tradition in nucleoside chemistry and the replacement of the 2′ or 3′ hydroxyl group of a nucleoside with a fluorine atom causes only a minor change in the overall structure, but significantly affects the stereoelectronic properties of the sugar moiety (Thibaudeau et al., 1998). It has been reported that important factors in the substitution of fluorine for hydrogen are the comparable size of the two atoms and the powerful electron withdrawing properties of fluorine relative to hydrogen, as well as the increased stability of the carbon-fluorine bond relative to the carbon-hydrogen bond. Hence, replacement of hydrogen by fluorine in a bioactive molecule is expected to cause minimal steric perturbations with respect to the molecule’s mode of binding to receptors or enzymes (Pitzer, 1960). Moreover, replacement of the hydrogen by fluorine causes not only changes in biological activity, but also increases the chemical and metabolic stability of nucleosides. The conformation of the sugar moiety of these analogs is strongly affected by the presence of the fluorine substituent and is different from that of natural deoxynucleosides (Pankiewicz et al., 1992).
Nucleosides bearing fluorine or fluorinated substituents within the carbohydrate moiety have been widely used in biochemical research and therapeutic treatment (Meng and Qing, 2006; Hagmann, 2008; Kirk, 2008). However, to date only few fluorinated CK derivatives have been prepared and their biological activity tested in plants (Clemenceau et al., 1996; Doležal et al., 2007). Only recently, several 6-benzylaminopurines substituted with β-D-arabinose at the N9-position with similar structures were synthesized in our laboratory, and subsequently patented as powerful antisenescence compounds compared with BAP, but the activity of these compounds were only tested in a detached wheat leaves senescence bioassay (Patent No. US 10,100,077 B2, 2018). Here, we present a new class of N6-substituted-2′-deoxy-2′-fluoro-9-(β)-D-arabinofuranosylpurine derivatives which show not only high levels of antisenescence activity but also promise as seed priming agents due to their high efficiency as plant growth promotors and plant stress alleviators. Their mode of action as priming agents is also discussed.
Materials and Methods
General Synthesis of N6-Substituted-2′-Deoxy-2′-Fluoro-9-(β)-D-Arabinofuranosylpurine Derivatives
All the compounds presented here were prepared by a slightly modified one-step synthesis (Wan et al., 2005) of 9-(2′-deoxy-2′-fluoro-β-D-arabinofuranosyl)hypoxanthine with benzylamine or isopentenylamine hydrochloride as appropriate in the presence of BOP and DIPEA in DMF (Figure 2). Firstly 9-(2′-deoxy-2′-fluoro-β-D-arabinofuranosyl)hypoxanthine (200 mg, 1 equiv.) and BOP (396 mg, 1.2 equiv.) were mixed together in DMF (4 mL) and subsequently DIPEA (194 μL, 1.5 equiv.) and benzylamine (1–3) or isopentenylamine hydrochloride (4) (1.2 equiv.) as the last component were added. Each reaction mixture was stirred under an argon atmosphere in an oil bath at a temperature of 55–60°C for 24 h and the effectiveness of the reaction was checked by Thin-layer chromatography (TLC) (CHCl3/MeOH 4:1). The reaction mixture was evaporated using a vacuum rotary evaporator to give a specifically colored gel. The resulting residue was carefully purified by column chromatography (1 and 3) or by preparative HPLC (2 and 4) to give the desired product, which in some cases (1 and 3) could be crystallized from various solvents.
General Procedures
The chromatographic purity and mass spectra of the compounds described were characterized using the HPLC-PDA-MS method. Samples (10 μL of 3 × 10–5 M in 1% methanol) were injected onto a reverse-phased column (Symmetry C18, 5 μm, 150 mm × 2.1 mm; Waters, Milford, MA, United States) tempered at 25°C. Solvent (A) consisted of 15 mM ammonium formate adjusted to pH 4.0 and solvent (B) consisted of methanol. The flow-rate was set to 200 μL min–1. A binary gradient was used: 0 min, 10% of B; 24 min; 90% of B; 34 min; 90% of B; 45 min; 10% of B using a Waters Alliance 2695 Separations Module (Waters, Manchester, United Kingdom). Then the effluent was introduced to a Waters 2996 PDA detector (Waters, Manchester, United Kingdom) (scanning range 210–700 nm with 1.2 nm resolution) and a tandem mass analyzer Q-Tof micro Mass Spectrometer (Waters, Manchester, United Kingdom) with an electrospray. The cone voltage was set to 20 V. Exact mass was determined by QTOF-MS (Synapt G2-Si, Waters, United Kingdom) operating in positive ion mode and recorded as (M + H)+. Melting points were determined on a Büchi Melting Point B-540 apparatus and are uncorrected. 1H NMR spectra were analyzed on a Jeol 500 SS spectrometer operating at a temperature of 300 K and a frequency of 500.13 MHz. The samples were prepared by dissolving in DMSO-d6. Tetramethylsilane (TMS) was used as an internal standard. TLC was carried out using silica gel 60 WF254 plates (Merck). Purification via column chromatography was performed using silica gel Davisil R LC60A 40-63 micron.
HPLC-MS Purification
A preparative HPLC-MS chromatography machine (Agilent 1290 Infinity II) was used coupled to a UV-VIS detector with a mass LC/MSD detector (Agilent InfinityLab) and an Agilent Prep-C18 column (5 μm, 21.2 mm × 50 mm, Waters, Milford, MA, United States) to obtain the final products. Analyzed samples were dissolved in 50% MeOH before injection. The mobile phase was methanol (A):H2O (B) with a flow rate of 20 mL min–1 and linear gradients (0 min, 10% B; 0–12 min; 90% B) were used.
HRMS Conditions
Samples (5 μL) were characterized using the HPLC-PDA-MS method. They were injected onto a reversed-phase column (Symmetry C18, 5 μm, 150 mm × 2.1 mm; Waters, Milford, MA, United States) incubated at 40°C. Solvent A was 15 mM ammonium formate adjusted to pH 4.0. Solvent B was methanol. The following linear gradient was used at a flow rate of 250 μL min–1: 0 min, 10% B; 0–15 min, 90% B. The effluent was introduced to a DAD detector (scanning range 210–400 nm with 1.2 nm resolution) and then to an electrospray source (source temperature 150°C, desolvation temperature 550°C, capillary voltage 1 kV, cone voltage 25 V). Nitrogen was used as the cone gas (50 L h–1) and the desolvation gas (1000 L h–1). Data acquisition was performed in full-scan mode (50–1000 Da) with a scan time of 0.5 s and collision energy of 4 eV; argon was used as the collision gas (optimized pressure of 5 × 10–3 mbar). Analyses were performed in positive mode (ESI+), therefore protonated molecules (M + H)+ were collected in each MS spectrum. For exact mass determination experiments, external calibration was performed using lock spray technology and a mixture of leucine/encephalin (50 pg μL–1) in an acetonitrile and water (1:1) solution with 0.1% formic acid as a reference. Accurate masses were calculated and used to determine the elemental composition of the analytes with a fidelity better than 1.0 ppm.
Cytokinin Bioassays
Cytokinin bioassays, including Amaranthus, tobacco callus and senescence bioassays, were carried out as previously described by Holub et al. (1998), using BAP as a positive control for all three classical CK bioassays. Results were recorded to define the highest activities of the four compounds prepared. All of them were dissolved in 0.5% DMSO and tested at five concentrations (from 10–8 to 10–4 M).
Plant Phenotyping – Rosette Growth of Seedlings From Arabidopsis Hormoprimed Seeds
The four compounds synthesized were tested as priming agents under optimal and two different stress conditions. Arabidopsis seeds (Arabidopsis thaliana accession Col-0) were sterilized and germinated as described by Ugena et al. (2018). During germination the compounds were added at four different concentrations (from 10–7 to 10–4 M) to germination medium containing 0.5× MS (pH 5.7) supplemented with a gelling agent (0.6% Phytagel; Sigma-Aldrich, Germany). Three days after germination, seedlings of similar size were transferred under sterile conditions into 48-well plates (Jetbiofil, Guangzhou, China). One seedling was transferred to each well filled with 850 mL 1× MS medium (pH 5.7; supplemented with 0.6% Phytagel), without stress treatment (optimal conditions) or containing 100 mM NaCl (as salt stress) or 100 mM mannitol (as osmotic stress), and the plates were sealed with perforated transparent foil allowing gas and water exchange. Hormopriming of 10–8 M BAP was also used as positive control for all tested growth conditions.
The 48-well plates containing the transferred Arabidopsis seedlings were placed in an OloPhen platform,1 which uses the PlantScreenTM XYZ system installed in a growth chamber with a controlled environment, and cool-white LED and far-red LED lighting (Photon Systems Instruments, Brno, Czechia). The conditions were set to simulate a long day with a temperature regime of 22/20°C in a 16/8 h light/dark cycle, an irradiance of 120 μmol photons of PAR m2 s–1 and a relative humidity of 60%. The PlantScreenTM XYZ system consists of a robotically driven arm holding an RGB camera with customized lighting panel and growing tables. The XYZ robotic arm was automatically moved above the plates to take RGB images of single plates from the top. The imaging of each 48 well plate was performed twice per day (at 10 a.m. and 4 p.m.) for 7 days as described in Ugena et al. (2018). As outcome, the individual image of 48 Arabidopsis seedlings per variant (treatment vs. growth condition) as biological replicates were used for the analyzed phenotyping traits.
Different traits were determined from the RGB images: Arabidopsis rosette growth curves [as changes in the green area (Pixels)], relative (RGR) and absolute (AGR) growth rate and final rosette size. All these traits were then used to define the mode of action of the compound under test. Using the traits, the plant biostimulant characterization (PBC) index was determined as described by Ugena et al. (2018). The PBC index was calculated as the sum of the values obtained from each phenotyping trait calculated as the differences (as the log2 of the ratio in each case) between the controls and treatment variants (compound and concentration) under the same growth conditions.
Determination of Arabidopsis Rosette Color Indices
To estimate the greenness of the Arabidopsis seedlings, and changes in leaf color, three vegetation indices (NGRDI, VARI, and GLI), which have been shown to be correlated with plant biomass, nutrient status, or tolerance to abiotic stress (Gitelson et al., 2002; Perry and Roberts, 2008; Hunt et al., 2013), were used. The images captured on the seventh day of an Arabidopsis rosette growth assay subjected to HTS were segmented for the extraction of leaf rosettes using software described in our previous report (De Diego et al., 2017). The values corresponding to particular color channels (red, R; green, G; and blue, B) were then extracted for each pixel within the plant mask, and the vegetation indices were calculated as described by Ugena et al. (2018). Subsequently, indices representing particular seedlings were determined by calculating the mean values for each plant mask. The mean and the standard error (SE) values for each 48-well plate were then calculated and represented in a graph.
Plant Hormone Quantification
Four independent biological replicates consisted in four individual pools from 12 Arabidopsis seedlings per variant were collected for the hormonal analysis. After purification and extraction, the concentration of each analyte was calculated using the standard isotope dilution method (Rittenberg and Foster, 1940). Briefly, as the first step a micro solid-phase extraction (μSPE) based on StageTip (STop And Go Extraction Tip) technology was used to purify the plant tissue samples. The μSPE protocol used in CK extraction and purification was applied as described by Svačinová et al. (2012), whereas auxins and ABA were isolated as described by Pênčík et al. (2018). CKs were determined using ultra-high performance liquid chromatography-electrospray tandem mass spectrometry (an Acquity UPLC I-Class System coupled with a Xevo TQ-S MS, Waters). Quantification of auxins and ABA was performed and the concentration of each analyte was calculated using the standard isotope dilution method on a 1260 Infinity II system coupled with a 6495B Triple Quadrupole LC/MS system (Agilent Technologies).
Statistical Analysis
To assess differences between treatments (compound and concentration) values for each non-invasive trait extracted by means of image analysis, a non-parametric (Dunn’s test after Kruskal–Wallis’ test parametric) method and a parametric method (Tukey’s HSD test after two-way ANOVA) were applied using the packages multcomp, FSA, and agricolae in RStudio (Version 1.1.463 – 2009-2018 RStudio, Inc.). Multivariate statistical analyses, including heatmap and principal component (PC) analysis, were also performed in RStudio using the packages gplots, cluster, tidyverse, factoextra, heatmap.plus, ggpubr, factoextra, FactoMineR, and corrplot.
Results
Synthesis of Four N9-Substituted CK Derivatives With a Fluorinated Carbohydrate Moiety
In this work, a group of three N9-substituted aromatic or one isoprenoid CK derivatives with a fluorinated carbohydrate moiety were synthesized (Figure 1) and their biological activity was investigated. The compounds prepared were characterized by 1H NMR, elemental analysis, melting points, TLC and ESI + MS. The purity of the prepared derivatives was confirmed by high-performance liquid chromatography (HPLC-UV) (Table 1).
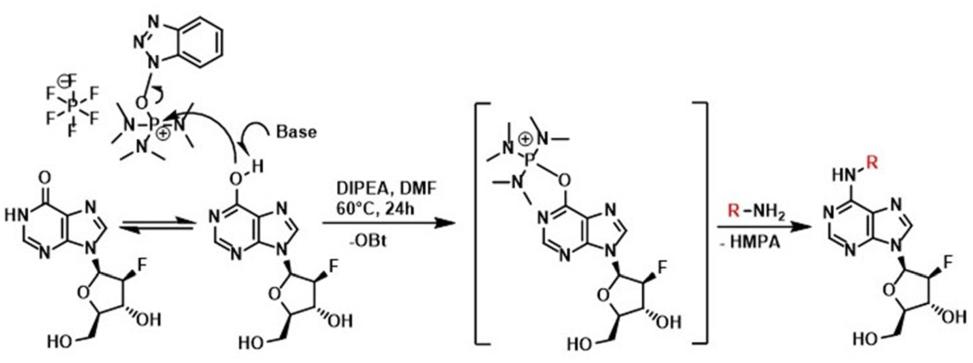
Figure 1. Scheme for the preparation of substituted 6-benzylamino-2′-deoxy-2-′fluoro-9-(β)-D-arabinofuranosylpurine derivatives by a method using Castro’s reagent and Hunig base.
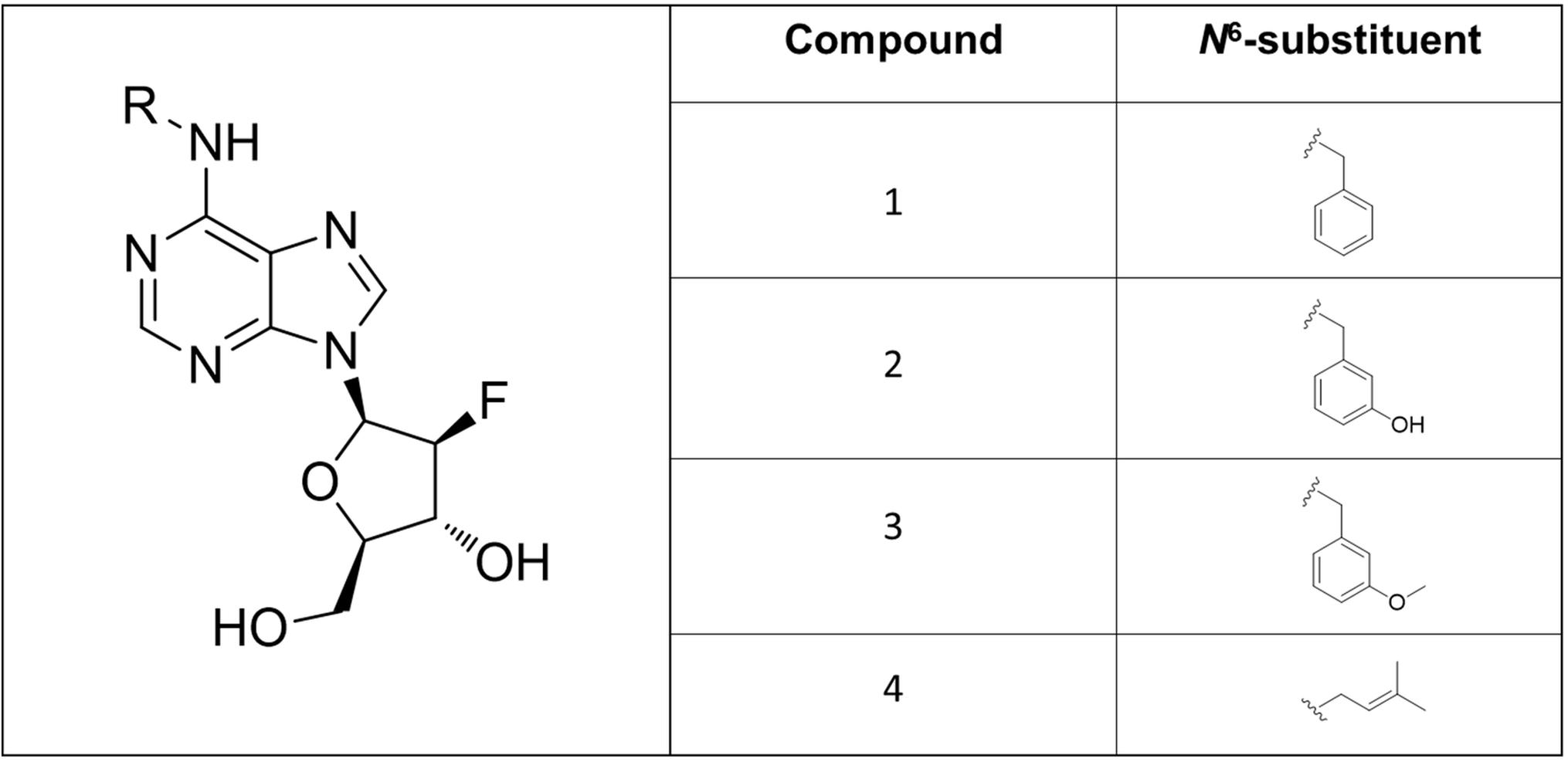
Figure 2. Structures of the newly synthesized 6-benzylamino-2′-deoxy-2-′fluoro-9-(β)-D-arabinofuranosylpurine derivatives.

Table 1. Physico-chemical properties of four new synthesized compounds: elemental analysis, melting temperature, mass of positively charged molecular ions analyzes by HPLC-MS, purity, and HRMS mass analysis.
First, the synthesis of a 2-fluoropentose from a pentoside precursor followed by its conversion into 9-(2-deoxy-2-fluoro-β-D-arabinofuranosyl) adenine was performed as reported in 1969 (Wright et al., 1969). Subsequently, 3-deoxy-3-fluoro-D-glucose was synthesized and converted into 2-deoxy-2-fluoro-D-arabinose via oxidation by sodium metaperiodate as described by Reichman et al. (1975). The compound 9-(2-deoxy-2-fluoro-β-D-arabinofuranosyl) adenine (F-ara-A) has previously been prepared by condensation of 6-chloropurine with 2-deoxy-2-fluoro-D-arabinofuranosyl bromide followed by conversion of the purine into adenine, but the reaction produced a mixture of four isomers and only a very low yield of the desired isomer could be isolated (Marquez et al., 1990). Later, a three-step synthesis of 9-(2-deoxy-2-fluoro-β-D-arabinofuranosyl)adenine was carried out via displacement of the 2′-hydroxyl group of 03′,05′,N6-tritrityladenosine and 03′,05′-ditritylinosine with diethylaminosulfur trifluoride, as published by Pankiewicz et al. (1992). The importance of introducing a fluorine at the 2′(S)(ara) site of purine deoxynucleosides has been highlighted, since 2′-deoxy-2′-fluoroarabinosides have been found to be biologically active and chemically stable against hydrolysis catalyzed both chemically and by purine nucleoside phosphorylase (Chu et al., 1989).
In the present study, all the aforementioned steps were followed and finally the synthesis of new compounds was performed as previously reported by Wan et al. (2005) with some modifications. Typically, the synthesis of purine nucleosides is based on the protection of hydroxyl groups, which prolongs this method to a four-step process with low yield. This transformation usually causes cleavage of the glycosyl bond, therefore only acid-labile protecting groups must be used. In our new simple one-step unprotected synthesis, BOP was used to activate the formation of a C-N bond. Subsequently, substitution by appropriate amines led to the formation of final products, after elimination of hexamethylphosphoramide (HMPA). However, the nucleophilic substitution of unprotected purine nucleosides with amines required longer reaction times compared with their protected counterparts (Wan et al., 2005).
CK-Like Activity of the New N9-Substituted CK Derivatives With a Fluorinated Carbohydrate Moiety in Cytokinin Bioassays
To evaluate the CK activities of the newly synthesized compounds, three classical in vitro CK bioassays were used. Despite the fact that all four of the new compounds are derived from CKs with known high levels of activity in all three bioassays, their 2′-deoxy-2′-fluoro-9-(β)-D-arabinofuranosyl purine derivatives showed decreases in activity in the Amaranthus and tobacco callus bioassays (Supplementary Table 1). On the other hand, high antisenescence activity was recorded in the bioassay based on evaluating the effect of the compound on retention of chlorophyll in excised wheat leaves kept in the dark (Table 2). The greatest ability to prevent chlorophyll degradation was shown by compounds 1 and 2, which reached, respectively, 277 and 267% of the values for the positive control BAP at concentrations of 10–4 M, followed by compound 3, which showed 179% of the BAP activity. Compound 4 had comparable activity to BAP (Table 2). Overall, these results showed that substitution at the N9 position with a fluorinated carbohydrate moiety selectively influences the CK-like activity, specifically improving the antisenescence properties of CKs modified in this way. This suggests that such CK analogs could activate plant processes related to stress responses and would therefore have antistress properties when applied to plants.
Priming With N9-Substituted CK Derivatives With a Fluorinated Carbohydrate Moiety Improves the Growth of Arabidopsis Under Both Optimal and Stress Conditions
To corroborate the involvement of these compounds in plant stress tolerance and better define the mode of action of our four newly synthesized compounds, we tested their effects on Arabidopsis growth and development under optimal and stress conditions using a complex multi-trait high-throughput screening approach (Ugena et al., 2018). The four compounds were used as seed priming agents at four concentrations (from 10–7 to 10–4 M). Non-primed and primed seeds were germinated under optimal conditions and then the seedlings were transferred into 48 well plates with 1× MS alone, or supplemented with 100 mM NaCl or 100 mM mannitol to induce salt or osmotic stress, respectively. First, we evaluated how the priming affected early seedling establishment. To do so, the rosette area of the seedlings transferred to control conditions (1× MS) at day 1 was determined. Here, we saw a clear interaction between compound and concentration affecting early seedling establishment (Figure 3 and Supplementary Figure 1). The seedlings developed from seeds primed with all the compounds except compound 1 had increased rosette area. The largest rosettes were observed after priming with the highest concentrations of compound 2 and 3, or lower concentrations of compound 4. Interestingly, priming with the highest concentration (10–4 M) of compound 4 caused strong growth inhibition, leading to seedlings reaching only half the size of the control (MOCK) seedlings (Figure 3 and Supplementary Figure 1).
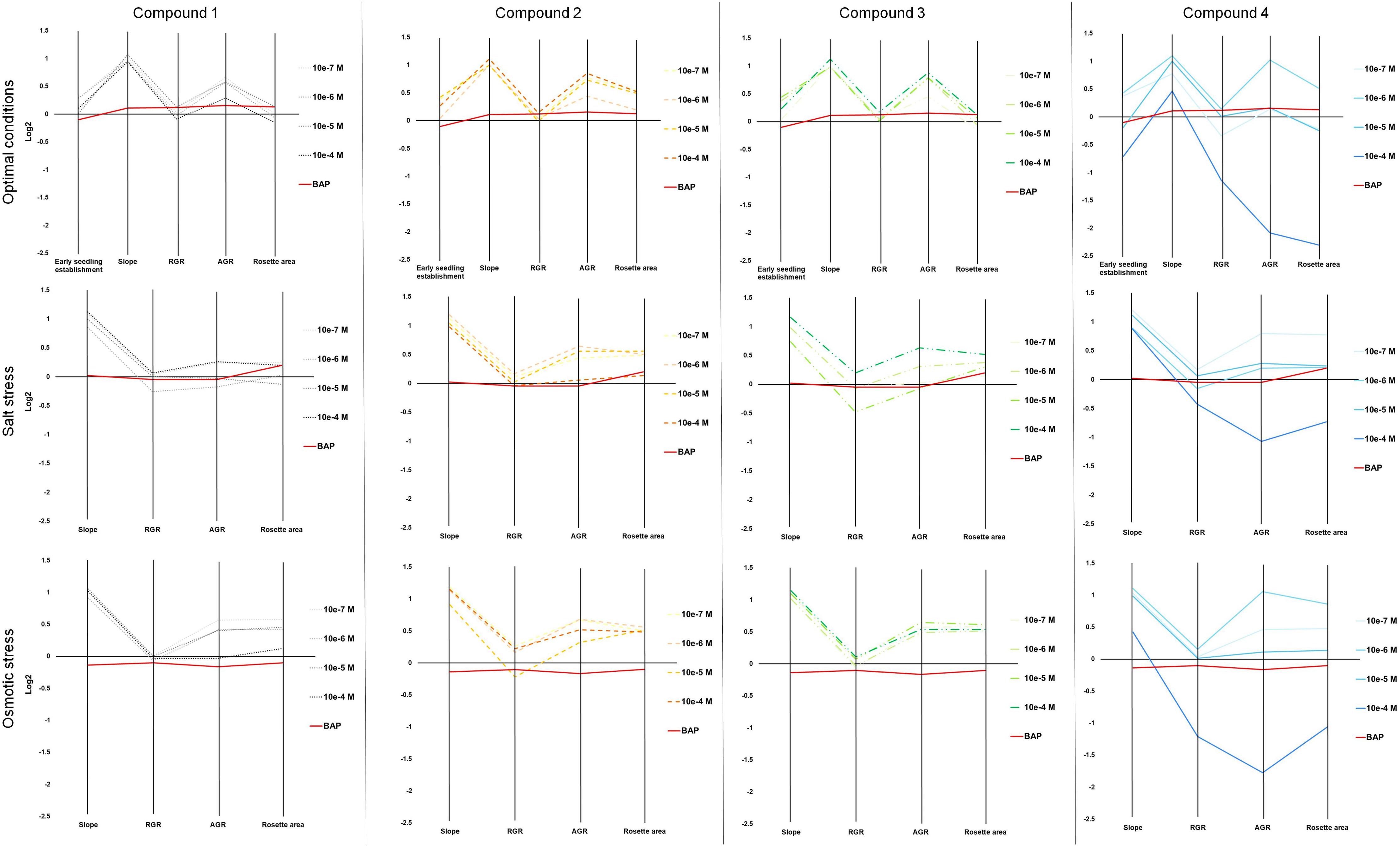
Figure 3. Parallel coordinate plot of the traits (Early seedling establishment, slope of the growing curve, RGR, AGR and the final rosette area) obtained from multi-trait high-throughput screening of Arabidopsis seedlings non-primed (MOCK) or primed with four different N9-substituted CK derivatives with a fluorinated carbohydrate moiety at four concentrations (10–7, 10–6, 10–5, and 10–4 M) and grown under optimal (upper panels), or salt (100 mM NaCl, middle panels) or osmotic (100 mM mannitol, bottom panels) stress conditions (N = 48). BAP at 10–8 M was used as positive control.
The rosette areas of the seedlings were further analyzed twice a day for an additional 6 days to record a growth curve (Supplementary Figure 2). All four compounds improved Arabidopsis seedling growth under control and stress conditions at some of the concentrations tested and there was significant interaction between compound concentration and growth conditions according to ANOVA. On the other hand, the highest concentration of compound 4 (10–4 M) showed inhibitory activity under all three of the conditions tested and the rosette areas were significantly reduced to 20, 60, and 48% of those in the non-primed control (MOCK) seedlings under, respectively, control, salt, and osmotic stress (Supplementary Figure 2).
Other traits such as relative growth rate (RGR) and absolute growth rate (AGR) were also calculated. For better visualization, these traits together with early seedling establishment and final rosette area (at day 7, Supplementary Figure 3) are presented in a parallel coordinate plot shown in Figure 3. To construct this, the differences between the controls and variants (compound and concentration) under the same growth conditions were calculated as the log2 of the ratio. The value obtained for each trait is shown in twelve independent parallel coordinate plots, one per compound (a total of 4) for optimal conditions, salt stress, and osmotic stress (Figure 3). Additionally, the priming effect of 10–8 BAP was evaluated as a positive control. Interestingly, in the parallel plot the three N9-substituted aromatic had similar profile whereas isoprenoid CK derivative showed different response (Figure 3). Under optimal growth conditions, priming with the new CK analogs improved some growth related traits analyzed (early seedling establishment, the slope of the curve, and AGR). At the assay end-point, mainly the seedlings primed with almost all concentrations of compound 2 had larger rosettes compared to the non-primed seedlings (MOCK) or those primed with the positive control (BAP) (Figure 3). These plants also presented higher homogeneity of the population (represented by coefficient of variance = standard deviation/Mean, %) compared with the MOCK variant (28.81 and 38.40, respectively) (Figure 4).
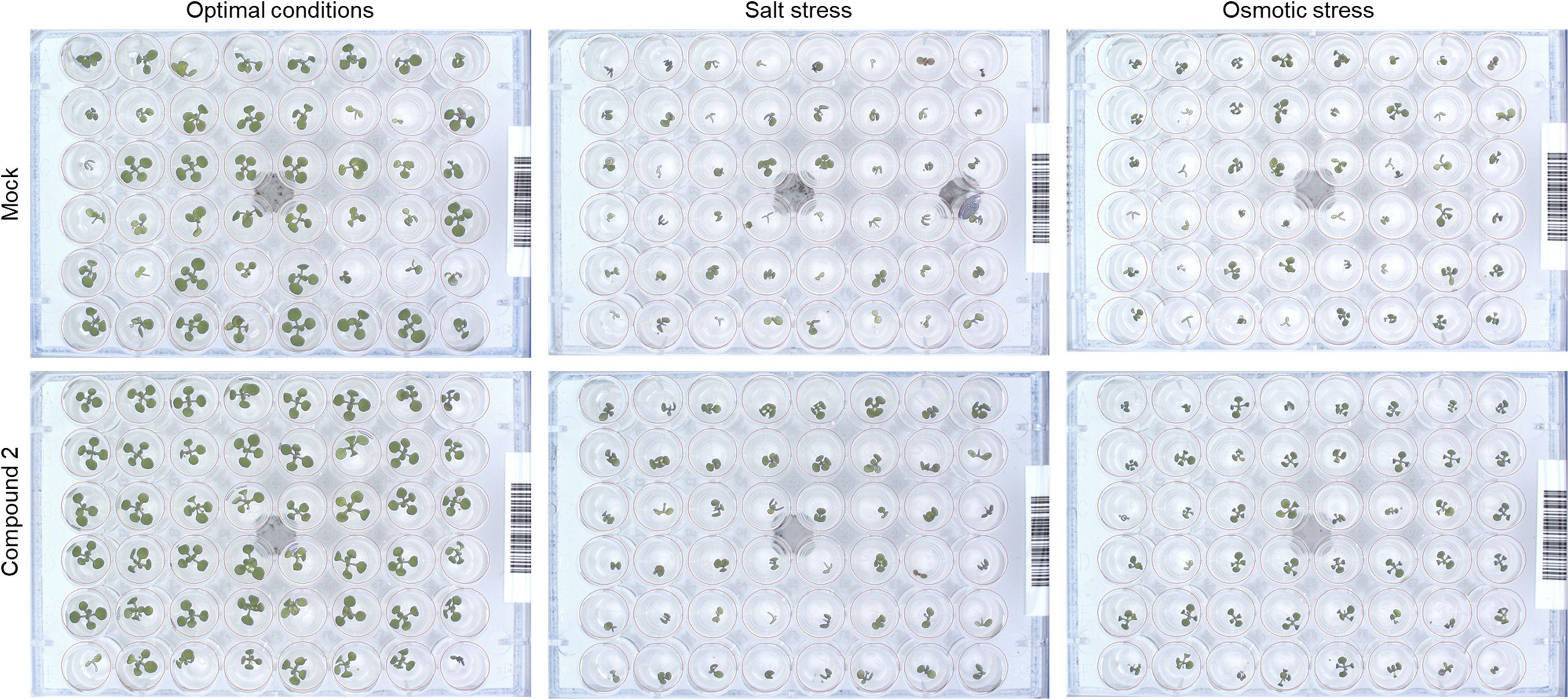
Figure 4. RGB images of the non-primed Arabidopsis seedlings (MOCK) and those primed with the best performing concentration of compound 2 (according to the PBC index, Table 3) grown under optimal growth condition (left images), salt (100 mM NaCl, middle images) or osmotic (100 mM mannitol, right images) stress.
Importantly, hormopriming improved the tolerance of the Arabidopsis seedlings to salt and mannitol induced stress by increasing the values of the slope of the curve, RGR, ARG and final rosette size compared to the negative and positive controls (Figure 3). In both cases, low concentrations of compound 4 (10–7 M for salt stress and 10–6 M for osmotic stress) resulted in the highest increases in the traits analyzed, whereas a concentration of 10–4 M inhibited plant growth under all growth conditions (Figure 3 and Supplementary Figures 1, 2). Conversely, plants primed with compound 2 showed improvements in all traits under both control and stress conditions (Figure 4). All these results were then combined to calculate the PBC index, which helps to simplify and sum up the overall outcomes in order to define the mode of action of a biostimulant (Ugena et al., 2018). As listed in Table 3, all compounds worked as plant growth promotors and stress alleviators at some of the concentrations tested, all with higher efficiency than the control CK BAP. The most efficient plant growth promotor was compound 4 followed by compound 2. However, whereas compound 2 improved growth at all concentrations tested and growth conditions (working as strong plant growth promotor and stress alleviator), compound 4 was highly toxic at the highest concentration (10–4 M), at which it showed a growth inhibitory effect (Table 3). Overall, we conclude that priming with the newly prepared N6-substituted-2′-deoxy-2′-fluoro-9-(β)-D-arabinofuranosylpurines had positive effects on Arabidopsis growth and, importantly, improved tolerance to salt and osmotic stress, with a stronger effect in the latter case (Figures 3, 4 and Table 3).
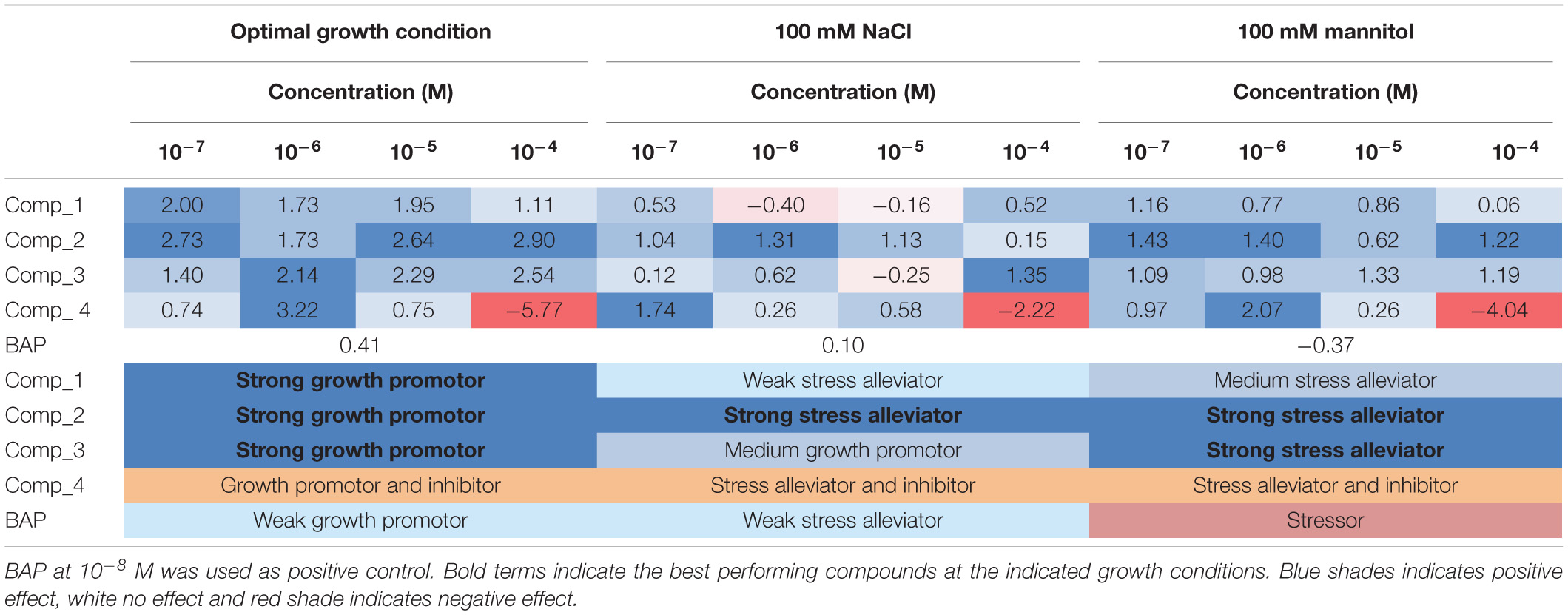
Table 3. Plant biostimulant characterization (PBC) index calculated by summing the relative changes (log2) obtained for the parallel coordinate plot (Figure 4) for each synthetized compound (four different N9-substituted CK derivatives with a fluorinated carbohydrate moiety) at four concentration (10–7, 10–6, 10–5, and 10–4 M) and growth condition; optimal, salt stress (100 mM NaCl), or osmotic stress (100 mM mannitol) (N = 48).
Hormopriming With N6-Substituted-2′-Deoxy-2′-Fluoro-9-(β)-D-Arabinofuranosylpurines Maintains Seedling Greenness
To gain a further understanding of priming with compound 2 (N6-substituted-2′-deoxy-2′-fluoro-9-(β)-D-arabinofuranosylpurines), changes in seedling color after 7 days under different growth conditions (optimal, salt, or osmotic stress) were determined. The degradation of chlorophyll, manifested as a change in Arabidopsis rosette color, represents one of the most important symptoms of stress (Ugena et al., 2018). Three different indices (NGRDI, VARI, and GLI) were calculated and presented in Figure 5. Significant differences were observed between seedlings from non-primed and primed seeds, especially regarding NGRDI and VARI indices under all growth conditions (Figure 4). Under optimal conditions, the highest values were obtained when the compound 2 was applied at 10–4 M, a concentration that also resulted in the highest PBC index (Table 3). However, under salt and osmotic stress, the highest NGRDI and VARI indices were observed when 10–5 M and 10–6 M were used (Figure 5). Taken together, these results corroborated the aforementioned antisenescence effect of this compound observed in the CK-like bioassays (Table 2).
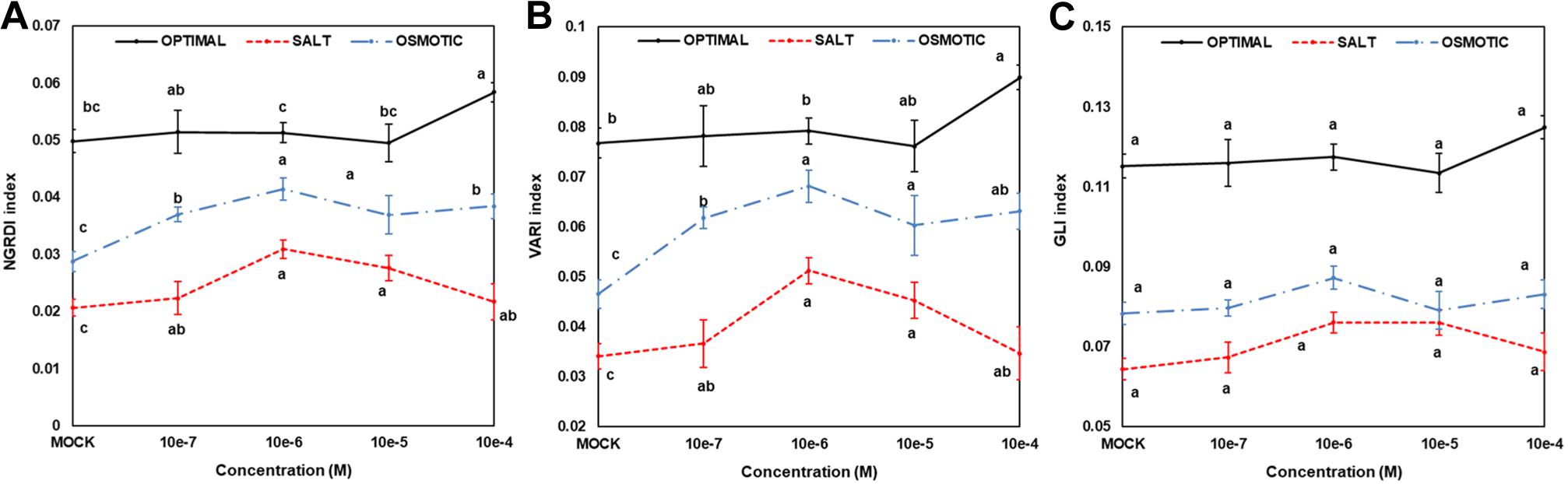
Figure 5. Color indices [NGRDI (A), VARI (B), or GLI (C)] used as greenness parameters for Arabidopsis seedlings from non-primed (MOCK) seeds or seeds primed with compound 2 at four concentrations (10–7, 10–6, 10–5, and 10–4 M) grown under optimal (upper panel), or salt (100 mM NaCl, middle panel), or osmotic (100 mM mannitol, bottom panel) stress conditions for 7 days (N = 48). Different letters mean significant differences among treatments (priming effect) for each growth conditions according to Dunn’s test after Kruskal–Wallis’ test.
Hormopriming With N6-Substituted-2′-Deoxy-2′-Fluoro-9-(β)-D-Arabinofuranosylpurines Improves Arabidopsis Growth and Stress Tolerance by Altering the Hormonal Profile
To understand the molecular nature of the mode of action of priming by the N6-substituted-2′-deoxy-2′-fluoro-9-(β)-D-arabinofuranosylpurines, the hormonal profile of Arabidopsis seedlings primed with the best performing compound 2 was analyzed at the end of the phenotyping experiment. The endogenous levels of CKs (Table 4), some auxins and ABA were quantified using LC/MS (Supplementary Table 2). For better visualization and interpretation, all metabolites were analyzed together using a heatmap (Figure 6A). The results separated the variants (treatments and growth conditions) into two clusters using the Spearman correlation as the distance method: one for the plants grown under salt stress and the control for osmotic stress, and a second cluster for the rest. Additionally, the first group was separated into two subclusters in which all plants grown under salt stress showed, in general, a reduction in content of the CK nucleotides, total auxin, and ABA (Figure 6A). On the other hand, the variants represented in the second cluster showed increased levels of these metabolites and of some N- and O-glucosides (DHZ7G, DHZ9G, tZ7G, and tZ9G), and IAA conjugated with glucose (IAAGlu). On the other hand, they reduced content of total CKs, bases, and ribosides, especially in the case of the hormoprimed seedlings under optimal and osmotic stress conditions (Figure 6A). Similar results were obtained when the distance among variants was determined (Supplementary Figure 4), in which the hormopriming seedlings grown under optimal and osmotic stress were separated by a short distance (close to 0; similar behavior), but the distance was longer for the primed plants grown under salt stress conditions.
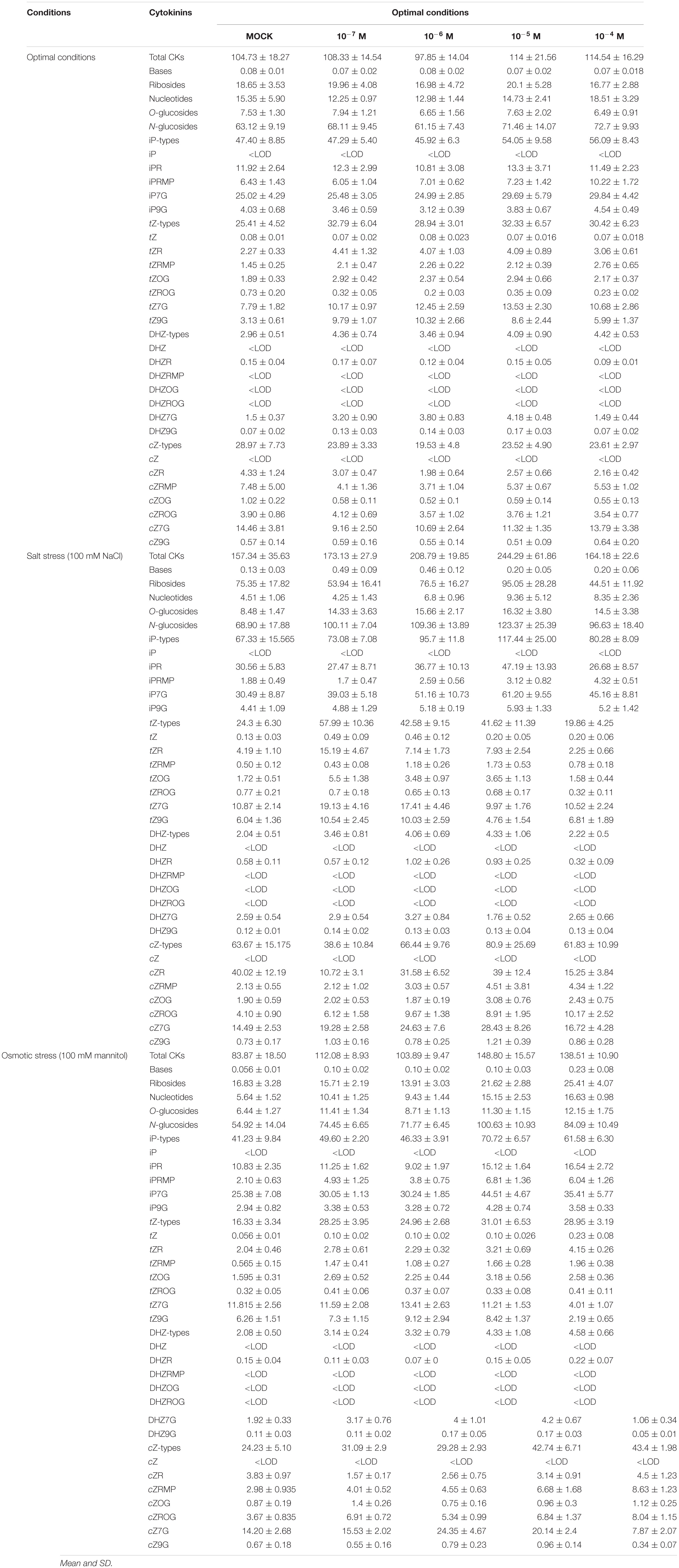
Table 4. Changes in CK levels (pmol g–1 FW) of 10-day-old Arabidopsis thaliana seedlings from non-primed seeds or seeds hormoprimed with compound 2 grown at four different concentrations (10–7, 10–6, 10–5, or 10–4 M) under optimal conditions, salt stress (100 mM NaCl), or osmotic stress (100 mM mannitol) for 7 days.
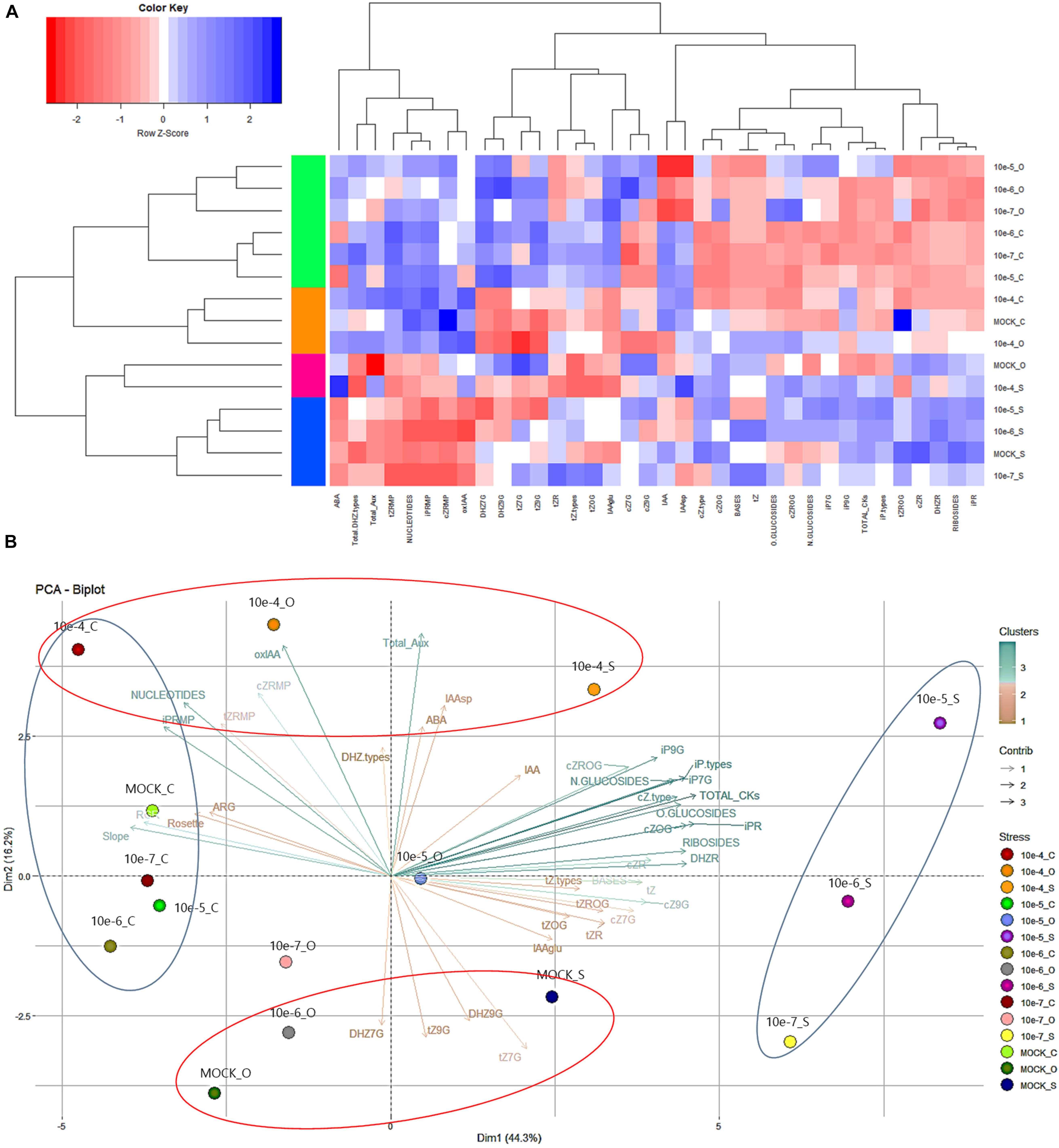
Figure 6. Heatmap (A) representing the changes in CKs, auxins, and ABA for Arabidopsis seedlings from non-primed (MOCK) seeds or seeds primed with compound 2 at four concentrations (10–7, 10–6, 10–5, and 10–4 M) grown under optimal (black line), or salt (100 mM NaCl, red line) or osmotic (100 mM mannitol, blue line) stress conditions for 7 days. Principal component analysis (Dimension, Dim) (B) of the same Arabidopsis seedlings.
To extend the analysis, a PC analysis was also performed (Figure 6B). The components PC1 and PC2 accounted for 60.5% of the total variance of the model. In PC1, there was clear evidence of contrasting behavior between all plants grown under optimal conditions and hormoprimed seedlings under salinity stress (blue ellipses). Thus, whereas the first group was positively correlated with the phenotyping traits and the CK nucleotides (synthesized de novo), which also showed a strong relationship (Supplementary Figure 5), the second group had a higher content of total CKs due to an increase in ribosides and N-glucosides (iP7G and iP9G) and O-Glucosides (cZOG and cZROG) (Figure 6B). Interestingly, hormopriming with the highest concentration (10–4 M) of compound 2 (as shown by PC2, red ellipses) induced similar contents of total auxins and the degradation form 2-oxindole-3-acetic acid (oxIAA) independent of growth conditions (Figure 6B), a pattern opposite to that in the MOCK variant under salt and osmotic stress. Overall, we demonstrated that in general hormopriming with the N6-substituted-2′-deoxy-2′-fluoro-9-(β)-D-arabinofuranosylpurines presented here induced changes in the hormonal content of Arabidopsis seedlings, thus conditioning the final phenotype, with the changes depending on the concentration of the compound and on growth conditions.
Discussion
In this work, a group of four N9-substituted CK derivatives with a fluorinated carbohydrate moiety (three with an aromatic and one with isoprenoid N6 side chain) were synthesized (Wan et al., 2005) by a slightly modified one-step reaction of 9-(2′-deoxy-2′-fluoro-β-D-arabinofuranosyl)hypoxanthine with the appropriate amine or amine hydrochloride in the presence of BOP and DIPEA in DMF (Figure 1 and Table 1). Nucleosides bearing fluorine or fluorinated substituents within the carbohydrate moiety have been used successfully in many biochemical research studies and therapeutic treatments. As an example, the ability of 9-(2-deoxy-β-D-arabinofuranosyl)adenine to completely inhibit the protozoan parasite Trichomonas vaginalis (Shokar et al., 2012), as well as its antibacterial (Gao et al., 2015) and antitrypanosomal (Ranjbarian et al., 2017) effect, have been reported. Significant antiviral activity was also confirmed for their dideoxy analogs. Montgomery et al. (1992) proved that 2-fluoro-9-(2,3-dideoxy-2-fluoro-β-D-arabinofuranosyl)adenine had anti-HIV properties, and 9-(2,3-dideoxy-2-fluoro-β-D-arabinofuranosyl)adenine was identified as an anti-HBV agent (Maruyama et al., 1999). However, their effects on plant species have never been investigated. Only few works presenting positive bioassay results for kinetin and isopentenyladenine analogs with N9-substituted with short aliphatic chains been published (Mik et al., 2011a, b). Similar to these results, our newly synthesized compounds (especially compound 2) also showed high levels of antisenescence activity. Moreover, the low activity of the N6-substituted-2′-deoxy-2′-fluoro-9-(β)-D-arabinofuranosylpurine derivatives in the callus bioassay confirms that fluorination of the sugar moiety prevents its hydrolysis to free bases and makes these compounds metabolically stable. Due to the strong effect of the CK analogs with a fluorinated carbohydrate moiety on the retention of chlorophyll in excised wheat leaves in the dark (Table 2), we hypothesized that the compounds would have antistress properties and analyzed their mode of action and their potential use as priming agents.
For many years, it has been shown that seed priming with certain hormones or other compounds improves seed germination and fitness in many plants (Van Hulten et al., 2006; Hussain et al., 2016). Seed priming improves stress tolerance through “priming memory,” which is established during priming and can be recruited later when seeds are exposed to stresses during germination (Chen and Arora, 2013). The beneficial effect of seed priming with CKs has been previously described under a range of growth conditions for many plant species, such as spring wheat (Triticum aestivum L.) (Iqbal and Ashraf, 2005; Iqbal et al., 2006) or basil (Ocimum basilicum L) (Bagheri et al., 2014). Despite this, there is not always a clear positive effect of priming, and it may also have a negative effect (Miyoshi and Sato, 1997; Sneideris et al., 2015; Willams et al., 2016), depending on the type of compound, the concentration used for priming, or the plant species and cultivars tested (reviewed by De Diego and Spíchal, 2020). In this work, hormopriming with the new N9-substituted CK derivatives improved early seed establishment and plant growth in A. thaliana under optimal and stress growth conditions (Figure 3), mainly by making the population more homogeneous, maintaining plant greenness (less chlorophyll degradation) and better nutrient status as defined by higher color indices (Figure 5). However, this response was concentration dependent. The best-performing compound was 6-(3-hydroxybenzylamino)-2′-deoxy-2′-fluoro-9-(β)-D-arabinofuranosylpurine, which was a good growth promotor under optimal growth conditions and a stress alleviator under both salt and osmotic stress at almost all concentrations tested, according to the PBC index (Table 3). As an exception, compound 4 at 10–4 M showed a strong growth inhibitory (toxic) effect. However, lower concentrations (10–7 or 10–6 M) improved plant growth under different growth conditions (Table 3). This underlines the importance of testing chemicals over broad concentration ranges and under different growth conditions. This is possible through initial high-throughput approaches using model plants such as Arabidopsis, followed by studies in the targeted species and specific growth conditions (Rouphael et al., 2018).
To understand better how these new compounds modify plant metabolism when they are used as priming agents, the endogenous levels of some plant hormones (CK, auxins, and ABA) were quantified. It was clear that hormopriming with compound 2 disrupted the plants’ hormonal homeostasis (Figure 6B). However, the changes varied depending on the conditions under which the plants were grown. Thus, different behaviors were observed between hormoprimed seedlings under optimal and osmotic stress conditions, and those under salt stress (Supplementary Figure 4). For example, under optimal conditions, primed Arabidopsis seedlings accumulated higher levels of ribotides (precursors), which were positively correlated with phenotypic traits such as AGR, RGR, slope of the growing curve and final rosette area (Supplementary Figure 5A). However, primed plants grown under salt stress conditions elevated their total CK content by increasing the amounts of conjugated forms including ribosides (iPR. DHZR and cZR), O-glucosides (cZOG and cZROG), and N-glucosides (iP7G and iP9G). It has been reported that riboside accumulation under stress conditions can be a defense mechanism, helping plants to deal with stress (Veerasamy et al., 2007; Man et al., 2011; De Diego et al., 2015). This may be because they play a crucial role in CK-mediated leaf longevity, and hence senescence, through phosphorylation of the CK response regulator ARR2 (reviewed by Hönig et al., 2018). For several years the cZ-type CKs and the base cZ were considered to be low-activity forms. However, in recent years, it has been proved that cZ-type CKs play important roles during plant development and in environmental interactions (Schäfer et al., 2015; Lacuesta et al., 2018). Thus, in primed Arabidopsis plants high levels of accumulation of cZOG and cZROG could be a strategy for maintaining plant growth under salt stress conditions. In support of this, it has been reported that the content of cZ-type CKs changes rapidly during maize seedling growth, and that cZ catabolism and glycosylation by cZ O-glucosyl transferases work synergistically to fine-tune cZ levels during plant development (Zalabák et al., 2014). Finally, in these primed plants there was also considerable accumulation of iP7G and iP9G. These two iP derivates are the terminal products of iP metabolism (Hošek et al., 2020). The iP metabolites including iP-N9G are the least active CKs, which seem not to be hydrolyzed and simply accumulate in the tissue (if not degraded by CKX) with no physiological effects (Hoyerová and Hošek, 2020). Overall, it is clear that priming with CK analogs modifies CK metabolism, but these changes are dependent on plant growth conditions. The results also pointed to the iP-type and cZ-type CKs as the main metabolites regulating the alleviation of salt stress in primed Arabidopsis seedlings.
Regarding auxins, levels of oxIAA mainly increased when compound 2 was applied at a high concentration (10–4 M) (Figure 6 and Supplementary Table 2). In recent years it has been proved that oxidizing IAA into oxIAA is of major physiological significance in the regulation of plant growth and development (Stepanova and Alonso, 2016). However, changes in other auxin-related metabolites did not show any correlation with the phenotypical changes in plants primed with compound 2.
Conclusion
In summary, in this case study we showed that hormopriming with N9-substituted CK derivatives with a fluorinated carbohydrate moiety seems to be a promising biotechnological approach for improving early seedling establishment and plant growth under both control and stress conditions. This is due to changes in plant hormone metabolism (especially of CKs and auxins) that differs according to growth conditions. Moreover, we believe that we have shown here that a complex approach is needed for selection of suitable compounds, by employing strategies allowing simultaneous testing of a broad range of concentrations and different growth conditions to define the conditions in which they are most efficient as priming agents.
Data Availability Statement
The original contributions presented in the study are included in the article/Supplementary Material, further inquiries can be directed to the corresponding author/s.
Author Contributions
MB and KD synthesized the compounds. AH, AEH, LS, and ND designed and performed the phenotyping experiments. AH, AP, and ON carried out the metabolite quantification. AH and ND performed the data analysis. MB, AH, LS, KD, and ND wrote the manuscript. All authors discussed the results.
Funding
This work was funded by the project “Plants as a tool for sustainable global development” (registration number: CZ.02.1.01/0.0/0.0/16_019/0000827) within the program Research, Development and Education (OP RDE) and the Internal Grant Agency of Palacký University (IGA_PrF_2020_010).
Conflict of Interest
The authors declare that the research was conducted in the absence of any commercial or financial relationships that could be construed as a potential conflict of interest.
Acknowledgments
We thank sees-editing for the English revision.
Supplementary Material
The Supplementary Material for this article can be found online at: https://www.frontiersin.org/articles/10.3389/fpls.2020.599228/full#supplementary-material
Supplementary Figure 1 | Early seedling establishment of Arabidopsis seedlings non-primed (MOCK) or primed with four different N9-substituted CK derivatives each with a fluorinated carbohydrate moiety at four concentrations (10–7, 10–6, 10–5, or 10–4 M) grown under control conditions (N = 48). Mean ± SE. Different letters mean significant differences among variants according to Tukey’s HSD test after ANOVA.
Supplementary Figure 2 | Growth curves for Arabidopsis seedlings non-primed (MOCK) or primed with four different N9-substituted CK derivatives each with a fluorinated carbohydrate moiety at four concentrations (10–7, 10–6, 10–5, or 10–4 M) grown for 7 days under optimal, salt stress (100 mM NaCl), or osmotic stress (100 mM mannitol) conditions (N = 48). Mean ± SE.
Supplementary Figure 3 | Maximum rosette size of Arabidopsis seedlings non-primed (MOCK) or primed with four different N9-substituted CK derivatives each with a fluorinated carbohydrate moiety at four concentrations (10–7, 10–6, 10–5, or 10–4 M) grown for 7 days under optimal, salt stress (100 mM NaCl), or osmotic stress (100 mM mannitol) conditions (N = 48). Mean ± SE. Different letters mean significant differences among variants according to Tukey’s HSD test after ANOVA.
Supplementary Figure 4 | Distance between Arabidopsis seedlings non-primed (MOCK) or primed with compound 2 at four concentrations (10–7, 10–6, 10–5, or 10–4 M) grown for 7 days under optimal (C), salt stress (100 mM NaCl, S) or osmotic stress (100 mM mannitol, O) conditions.
Supplementary Figure 5 | Correlation matrix (A) and contribution of the loadings to each PC (Dim) (B) according to multivariate statistical analyses of traits and metabolites in Arabidopsis seedlings non-primed (MOCK) or primed with compound 2 at four concentrations (10–7, 10–6, 10–5, or 10–4 M) grown for 7 days under optimal, salt stress (100 mM NaCl), or osmotic stress (100 mM mannitol) conditions (N = 48).
Abbreviations
BAP, 6-benzylaminopurine, ABA, abscisic acid, CKs, cytokinins, IAA, indole-3-acetic acid, iP, N6-isopentenyladenine, cZ, cis-zeatin, DHZ, dihydrozeatin, tZ, trans-zeatin, HTS, high-throughput screening, PBC, plant biostimulant characterization index.
Footnotes
References
Bagheri, A., Bagherifard, A., Saborifard, H., Ahmadi, M., and Safarpoor, M. (2014). Effects drought, cytokinins and GA3 on seedling growth of Basil (Ocimum basilicum). Int. J. Adv. Biol. Biomed. Res. 2, 489–493. doi: 10.1017/CBO9781107415324.004
Bairu, M. W., Jain, N., Stirk, W. A., Doležal, K., and Van Staden, J. (2009). Solving the problem of shoot-tip necrosis in Harpagophytum procumbens by changing the cytokinin types, calcium and boron concentrations in the medium. South Afr. J. Bot. 75, 122–127. doi: 10.1016/j.sajb.2008.08.006
Bielach, A., Hrtyan, M., and Tognetti, V. B. (2017). Plants under stress: involvement of auxin and cytokinin. Int. J. Mol. Sci. 18:1427. doi: 10.3390/ijms18071427
Brzobohatý, B., Moore, I., Kristoffersen, P., Bako, L., Campos, N., and Schell, J. (1993). Release of active cytokinin by a beta-glucosidase localized to the maize root meristem. Science 262, 1051–1054. doi: 10.1126/science.8235622
Chen, K., and Arora, R. (2013). Priming memory invokes seed stress-tolerance. Environ. Exp. Bot. 94, 33–45. doi: 10.1016/j.envexpbot.2012.03.005
Chu, C. K., Matulic-Adamic, J., Huang, J.-T., Chou, T.-C., Burchanal, J. H., Fox, J. J., et al. (1989). Nucleotides. CXXXV. Synthesis of some 9-(2-Deoxy-2-fluoro-(β-D-arabinofuranosyl)-9H-purines and their biological activities. Chem. Pharm. Bull. 37, 336–339.
Clemenceau, D., Cousseau, J., Martin, V., Molines, H., Wakselman, C., Mornet, R., et al. (1996). Synthesis and cytokinin activity of two fluoro derivatives of N6-isopentenyladenine. J. Agric. Food Chem. 44, 320–323. doi: 10.1021/jf9501148
Conrath, U. (2011). Molecular aspects of defence priming. Trends Plant Sci. 16, 524–531. doi: 10.1016/J.TPLANTS.2011.06.004
Criado, M. V., Caputo, C., Roberts, I. N., Castro, M. A., and Barneix, A. J. (2009). Cytokinin-induced changes of nitrogen remobilization and chloroplast ultrastructure in wheat (Triticum aestivum). J. Plant Physiol. 166, 1775–1785. doi: 10.1016/j.jplph.2009.05.007
De Diego, N., Fürst, T., Humplík, J. F., Ugena, L., Podlešáková, K., and Spíchal, L. (2017). An automated method for high-throughput screening of Arabidopsis rosette growth in multi-well plates and its validation in stress conditions. Front. Plant Sci. 8:1702. doi: 10.3389/fpls.2017.01702
De Diego, N., Saiz-Fernández, I., Rodríguez, J. L., Pérez-Alfocea, P., Sampedro, M. C., Barrio, R. J., et al. (2015). Metabolites and hormones are involved in the intraspecific variability of drought hardening in radiata pine. J. Plant Physiol. 188, 64–71. doi: 10.1016/j.jplph.2015.08.006
De Diego, N., and Spíchal, L. (2020). “Use of plant metabolites to mitigate stress effects in crops,” in The Chemical Biology of Plant Biostimulants, eds D. Geelen and L. Xu (Hoboken, NJ: Wiley), 261–300. doi: 10.1002/9781119357254.ch11
Doležal, K., Popa, I., Hauserová, E., Spíchal, L., Chakrabarty, K., Novák, O., et al. (2007). Preparation, biological activity and endogenous occurrence of N6-benzyladenosines. Bioorganic Med. Chem. 15, 3737–3747. doi: 10.1016/j.bmc.2007.03.038
Frébort, I., Kowalska, M., Hluska, T., Frébortová, J., and Galuszka, P. (2011). Evolution of cytokinin biosynthesis and degradation. J. Exp. Bot. 62, 2431–2452. doi: 10.1093/jxb/err004
Gamir, J., Sánchez-Bel, P., and Flors, V. (2014). Molecular and physiological stages of priming: how plants prepare for environmental challenges. Plant Cell Rep. 33, 1935–1949. doi: 10.1007/s00299-014-1665-9
Gao, J., Li, W., Niu, L., Cao, R., and Yin, W. (2015). Isolation and structural elucidation of novel antimicrobial compounds from maggots of Chrysomyis megacephala Fabricius. Nat. Prod. Res. 29, 239–246. doi: 10.1080/14786419.2014.948875
George, E. F., Hall, M. A., and De Klerk, G. J. (2008). “Plant growth regulators II: cytokinins, their analogues and antagonists,” in Plant Propagation by Tissue Culture, 3rd Edn, eds E. F. George, M. A. Hall, and G. J. D. Klerk (Dordrecht: Springer). doi: 10.1007/978-1-4020-5005-3_6
Gitelson, A. A., Kaufman, Y. J., Stark, R., and Rundquist, D. (2002). Novel algorithms for remote estimation of vegetation fraction. Remote Sens. Environ. 80, 76–87. doi: 10.1016/S0034-4257(01)00289-9
Hagmann, W. K. (2008). The many roles for fluorine in medicinal chemistry. J. Med. Chem. 51, 4359–4369. doi: 10.1021/jm800219f
Holub, J., Hanuš, J., Hanke, D. E., and Strnad, M. (1998). Biological activity of cytokinins derived from Ortho–and Meta-Hydroxybenzyladenine. Plant Growth Regul. 26, 109–115.
Hönig, M., Plíhalová, L., Husičková, A., Nisler, J., and Doležal, K. (2018). Role of cytokinins in senescence, antioxidant defence and photosynthesis. Int. J. Mol. Sci. 19, 1–23. doi: 10.3390/ijms19124045
Hošek, P., Hoyerová, K., Kiran, N. S., Dobrev, P. I., Zahajská, L., Filepová, R., et al. (2020). Distinct metabolism of N−glucosides of isopentenyladenine and trans−zeatin determines cytokinin metabolic spectrum in Arabidopsis. New Phytol. 225, 2423–2438. doi: 10.1111/nph.16310
Hoyerová, K., and Hošek, P. (2020). New insights into the metabolism and role of cytokinin N-glucosides in plants. Front. Plant Sci. 11:741. doi: 10.3389/fpls.2020.00741
Hunt, E. R., Doraiswamy, P. C., McMurtrey, J. E., Daughtry, C. S. T., Perry, E. M., and Akhmedov, B. (2013). A visible band index for remote sensing leaf chlorophyll content at the canopy scale. Int. J. Appl. Earth Obs. Geoinf. 21, 103–112. doi: 10.1016/J.JAG.2012.07.020
Hussain, S., Khan, F., Cao, W., Wu, L., and Geng, M. (2016). Seed priming alters the production and detoxification of reactive oxygen intermediates in rice seedlings grown under sub-optimal temperature and nutrient supply. Front. Plant Sci. 7:439. doi: 10.3389/fpls.2016.00439
Ibrahim, E. A. (2016). Seed priming to alleviate salinity stress in germinating seeds. J. Plant Physiol. 192, 38–46. doi: 10.1016/j.jplph.2015.12.011
Iqbal, M., and Ashraf, M. (2005). Presowing seed treatment with cytokinins and its effect on growth, photosynthetic rate, ionic levels and yield of two wheat cultivars differing in salt tolerance. J. Integr. Plant Biol. 47, 1315–1325. doi: 10.1111/j.1744-7909.2005.00163.x
Iqbal, M., Ashraf, M., and Jamil, A. (2006). Seed enhancement with cytokinins: changes in growth and grain yield in salt stressed wheat plants. Plant Growth Regul. 50, 29–39. doi: 10.1007/s10725-006-9123-5
Jisha, K. C., Vijayakumari, K., and Puthur, J. T. (2013). Seed priming for abiotic stress tolerance: an overview. Acta Physiol. Plant. 35, 1381–1396. doi: 10.1007/s11738-012-1186-5
Jordi, W., Schapendonk, A., Davelaar, E., Stoopen, G. M., Pot, C. S., De Visser, R., et al. (2000). Increased cytokinin levels in transgenic P(SAG12)-IPT tobacco plants have large direct and indirect effects on leaf senescence, photosynthesis and N partitioning. Plant Cell Environ. 23, 279–289. doi: 10.1046/j.1365-3040.2000.00544.x
Kirk, K. L. (2008). Fiuorination in medical chemistry: methods, strategies, and recent development. Org. Process Res. Dev. 12:305. doi: 10.1021/op700134j
Lacuesta, M., Saiz-Fernández, I., Podlešáková, K., Miranda-Apodaca, J., Novák, O., Doležal, K., et al. (2018). The trans and cis zeatin isomers play different roles in regulating growth inhibition induced by high nitrate concentrations in maize. Plant Growth Regul. 85, 199–209. doi: 10.1007/s10725-018-0383-7
Lutts, S., Benincasa, P., Wojtyla, L., Kubala, S., Pace, R., Lechowska, K., et al. (2016). “Seed priming: new comprehensive approaches for an old empirical technique,” in New Challenges in Seed Biology–Basic and Translational Research Driving Seed Technology, eds S. Araujo, and A. Balestrazzi (London: InTech). Available online at: https://www.intechopen.com/books/new-challenges-in-seed-biology-basic-and-translational-research-driving-seed-technology/seed-priming-new-comprehensive-approaches-for-an-old-empirical-technique. doi: 10.5772/64420
Man, D., Bao, Y. X., Han, L. B., and Zhang, X. (2011). Drought tolerance associated with proline and hormone metabolism in two tall fescue cultivars. HortScience 46, 1027–1032. doi: 10.21273/hortsci.46.7.1027
Marchetti, C. F., Škrabišová, M., Galuszka, P., Novák, O., and Causin, H. F. (2018). Blue light suppression alters cytokinin homeostasis in wheat leaves senescing under shading stress. Plant Physiol. Biochem. 130, 647–657. doi: 10.1016/j.plaphy.2018.08.005
Marquez, V. E., Tseng, C. K. H., Kelley, J. A., Ford, H., Roth, J. S., Driscoll, J. S., et al. (1990). Acid-stable 2′-fluoro purine dideoxynucleosides as active agents against HIV. J. Med. Chem. 33, 978–985. doi: 10.1021/jm00165a015
Maruyama, T., Takamatsu, S., Kozai, S., Satoh, Y., and Izawa, K. (1999). Synthesis of 9-(2-deoxy-2-fluoro-β-D-arabinofuranosyl)adenine bearing a selectively removable protecting group. Chem. Pharm. Bull. 47, 966–970. doi: 10.1248/cpb.47.966
Meng, W.-D., and Qing, F.-L. (2006). Fluorinated nucleosides as antiviral and antitumor agents. Curr. Top. Med. Chem. 6, 1499–1528. doi: 10.2174/156802606777951082
Mik, V., Szüčová, L., Šmehilová, M., Zatloukal, M., Doležal, K., Nisler, J., et al. (2011a). N9-substituted derivatives of kinetin: effective anti-senescence agents. Phytochemistry 72, 821–831. doi: 10.1016/j.phytochem.2011.02.002
Mik, V., Szüčová, L., Spíchal, L., Plíhal, O., Nisler, J., Zahajská, L., et al. (2011b). N9-Substituted N6-[(3-methylbut-2-en-1-yl)amino]purine derivatives and their biological activity in selected cytokinin bioassays. Bioorganic Med. Chem. 19, 7244–7251. doi: 10.1016/j.bmc.2011.09.052
Miller, C. O., Skoog, F., Von Saltza, M. H., and Strong, F. M. (1955). Kinetin, a cell division factor from deoxyribonucleic acid. J. Am. Chem. Soc. 77:1392. doi: 10.1021/ja01610a105
Miyoshi, K., and Sato, T. (1997). The effects of kinetin and gibberellin on the germination of dehusked seeds of indica and japonica rice (Oryza sativa L.) under anaerobic and aerobic conditions. Ann. Bot. 80, 479–483. doi: 10.1006/anbo.1997.0470
Montgomery, J. A., Shortnacy-Fowler, A. T., Clayton, S. D., Riordan, J. M., and Secrist, J. A. (1992). Synthesis and biological activity of 2’-fluoro-2-halo derivatives of 9-β-D-Arabinofuranosyladenine. J. Med. Chem. 35, 397–401. doi: 10.1021/jm00080a029
Pankiewicz, K. W., Krzeminski, J., Ciszewski, L. A., Ren, W. Y., and Watanabe, K. A. (1992). A synthesis of 9-(2-Deoxy-2-fluoro-β-D-arabinofuranosyl)adenine and hypoxanthine. An Effect of C3′-endo to C2′-endo conformational shift on the reaction course of 2′-hydroxyl group with DAST. J. Org. Chem. 57, 553–559. doi: 10.1021/jo00028a030
Paparella, S., Araújo, S. S., Rossi, G., Wijayasinghe, M., Carbonera, D., and Balestrazzi, A. (2015). Seed priming: state of the art and new perspectives. Plant Cell Rep. 34, 1281–1293. doi: 10.1007/s00299-015-1784-y
Pavlů, J., Novák, J., Koukalová, V., Luklová, M., Brzobohatý, B., and Černý, M. (2018). Cytokinin at the crossroads of abiotic stress signalling pathways. Int. J. Mol. Sci. 19, 1–36. doi: 10.3390/ijms19082450
Pênčík, A., Casanova-Sáez, R., Pilaøová, V., Žukauskaite, A., Pinto, R., Micol, J. L., et al. (2018). Ultra-rapid auxin metabolite profiling for high-throughput mutant screening in Arabidopsis. J. Exp. Bot. 69, 2569–2579. doi: 10.1093/jxb/ery084
Perry, E. M., and Roberts, D. A. (2008). Sensitivity of narrow-band and broad-band indices for assessing nitrogen availability and water stress in an annual crop. Agron. J. 100:1211. doi: 10.2134/agronj2007.0306
Pitzer, K. S. (1960). The nature of the chemical bond and the structure of molecules and crystals: an introduction to modern structural chemistry. J. Am. Chem. Soc. 82:4121. doi: 10.1021/ja01500a088
Plíhal, O., Szüčová, L., and Galuszka, P. (2013). N9-substituted aromatic cytokinins with negligible side effects on root development are an emerging tool for in vitro culturing. Plant Signal. Behav. 8:e24392. doi: 10.4161/psb.24392
Podlešáková, K., Zalabák, D., Čudejková, M., Plíhal, O., Szüčová, L., Doležal, K., et al. (2012). Novel cytokinin derivatives do not show negative effects on root growth and proliferation in submicromolar range. PLoS One 7:e39293. doi: 10.1371/journal.pone.0039293
Ranjbarian, F., Vodnala, M., Alzahrani, K. J. H., Ebiloma, G. U., De Koning, H. P., and Hofer, A. (2017). 9-(2’-Deoxy-2-Fluoro-β-D-Arabinofuranosyl) adenine is a potent antitrypanosomal adenosine analogue that circumvents transport-related drug resistance. Antimicrob. Agents Chemother. 61:e02719-16. doi: 10.1128/AAC.02719-16
Reichman, U., Watanabe, K. A., and Fox, J. J. (1975). A practical synthesis of 2-deoxy-2-fluoro-D-arabinofuranose derivates. Carbohydr. Res. 42, 233–240.
Rittenberg, D., and Foster, G. L. (1940). A new procedure for quantitative analysis by isotope dilution, with application to the determination of amino acids and fatty acids. R. Soc. Open Sci. 5:181322.
Roitsch, T., and Ehneß, R. (2000). Regulation of source / sink relations by cytokinins. Plant Growth Regul. 32, 359–367. doi: 10.1023/A:1010781500705
Rouphael, Y., Spíchal, L., Panzarová, K., Casa, R., and Colla, G. (2018). High-throughput plant phenotyping for developing novel biostimulants: from lab to field or from field to lab? Front. Plant Sci. 9:1197. doi: 10.3389/fpls.2018.01197
Sakakibara, H. (2006). CYTOKININS: activity, biosynthesis, and translocation. Annu. Rev. Plant Biol. 57, 431–449. doi: 10.1146/annurev.arplant.57.032905.105231
Savvides, A., Ali, S., Tester, M., and Fotopoulos, V. (2016). Chemical priming of plants against multiple abiotic stresses: mission possible? Trends Plant Sci. 21, 329–340. doi: 10.1016/j.tplants.2015.11.003
Schäfer, M., Brütting, C., Meza-Canales, I. D., Großkinsky, D. K., Vankova, R., Baldwin, I. T., et al. (2015). The role of cis-zeatin-type cytokinins in plant growth regulation and mediating responses to environmental interactions. J. Exp. Bot. 66, 4873–4884. doi: 10.1093/jxb/erv214
Shokar, A., Au, A., An, S. H., Tong, E., Garza, G., Zayas, J., et al. (2012). S-Adenosylhomocysteine hydrolase of the protozoan parasite Trichomonas vaginalis: potent inhibitory activity of 9-(2-deoxy-2-fluoro-β,d- arabinofuranosyl)adenine. Bioorganic Med. Chem. Lett. 22, 4203–4205. doi: 10.1016/j.bmcl.2012.03.087
Sneideris, L. C., Gavassi, M. A., Campos, M. L., D’amico-Damião, V., and Carvalho, R. F. (2015). Effects of hormonal priming on seed germination of pigeon pea under cadmium stress. An. Acad. Bras. Cienc. 87, 1847–1852. doi: 10.1590/0001-3765201520140332
Stepanova, A. N., and Alonso, J. M. (2016). Auxin catabolism unplugged: role of IAA oxidation in auxin homeostasis. Proc. Natl. Acad. Sci. U.S.A. 113, 10742–10744. doi: 10.1073/pnas.1613506113
Strnad, M. (1997). The aromatic cytokinins. Physiol. Plant 101, 674–688. doi: 10.1111/j.1399-3054.1997.tb01052.x
Svačinová, J., Novák, O., Plačková, L., Lenobel, R., Holík, J., Strnad, M., et al. (2012). A new approach for cytokinin isolation from Arabidopsis tissues using miniaturized purification: pipette tip solid-phase extraction. Plant Methods 8:17. doi: 10.1186/1746-4811-8-17
Thibaudeau, C., Plavec, J., and Chattopadhyaya, J. (1998). A new generalized Karplus-type equation relating vicinal proton-fluorine coupling constants to H-C-C-F Torsion Angles. J. Org. Chem. 63:4967. doi: 10.1021/jo980144k
Uddin, M. N., Hossain, M. A., and Burritt, D. (2016). “Salinity and drought stress: similarities and differences in oxidative responses and cellular redox regulation,” in Water Stress and Crop Plants: A Sustainable Approach, ed. P. Ahmad (Hoboken, NJ: Wiley), 86–101. doi: 10.1002/9781119054450.ch7
Ugena, L., Hýlová, A., Podlešáková, K., Humplík, J. F., Doležal, K., De Diego, N., et al. (2018). Characterization of biostimulant mode of action using novel multi-trait high-throughput screening of Arabidopsis germination and rosette Growth. Front. Plant Sci. 9:1327. doi: 10.3389/fpls.2018.01327
Van Hulten, M., Pelser, M., Van Loon, L. C., Pieterse, C. M. J., and Ton, J. (2006). Costs and benefits of priming for defense in Arabidopsis. Proc. Natl. Acad. Sci. U.S.A. 103, 5602–5607. doi: 10.1073/pnas.0510213103
Veerasamy, M., He, Y., and Huang, B. (2007). Leaf senescence and protein metabolism in creeping bentgrass exposed to heat stress and treated with cytokinins. J. Am. Soc. Hortic. Sci. 132, 467–472. doi: 10.21273/jashs.132.4.467
Wan, Z. K., Binnun, E., Wilson, D. P., and Lee, J. (2005). A highly facile and efficient one-step synthesis of N6-adenosine and N6-2′-deoxyadenosine derivatives. Org. Lett. 7, 5877–5880. doi: 10.1021/ol052424+
Werbrouck, S. P. O., van der Jeugt, B., Dewitte, W., Prinsen, E., Van Onckelen, H. A., and Debergh, P. C. (1995). The metabolism of benzyladenine in Spathiphyllum floribundum “Schott Petite” in relation to acclimatisation problems. Plant Cell Rep. 14, 662–665. doi: 10.1007/BF00232734
Werner, T., Holst, K., Pörs, Y., Guivarc’h, A., Mustroph, A., Chriqui, D., et al. (2008). Cytokinin deficiency causes distinct changes of sink and source parameters in tobacco shoots and roots. J. Exp. Bot. 59, 2659–2672. doi: 10.1093/jxb/ern134
Willams, F. S. B., Fábio, S., Leandro, C. M., de, O., Paulo, H., and Menezes, C. (2016). Comparison of seed priming techniques with regards to germination and growth of watermelon seedlings in laboratory condition. African J. Biotechnol. 15, 2596–2602. doi: 10.5897/ajb2016.15279
Wright, J. A., Taylor, N. F., and Fox, J. J. (1969). Nucleosides. LX. Fluorocarbohydrates. XXII. Synthesis of 2-Deoxy-2-fluoro-D-arabinose and 9-(2-Deoxy-2-fluoro-α- and -β-D-arabinofuranosyl)adenines. J. Org. Chem. 34, 2632–2636. doi: 10.1021/jo01261a031
Zalabák, D., Galuszka, P., Mrízová, K., Podlešáková, K., Gu, R., and Frébortová, J. (2014). Biochemical characterization of the maize cytokinin dehydrogenase family and cytokinin profiling in developing maize plantlets in relation to the expression of cytokinin dehydrogenase genes. Plant Physiol. Biochem. 74, 283–293. doi: 10.1016/j.plaphy.2013.11.020
Keywords: abiotic stress, antisenescence, Arabidopsis, cytokinin analogs, hormopriming, plant biostimulant characterization index
Citation: Bryksová M, Hybenová A, Hernándiz AE, Novák O, Pěnčík A, Spíchal L, De Diego N and Doležal K (2020) Hormopriming to Mitigate Abiotic Stress Effects: A Case Study of N9 -Substituted Cytokinin Derivatives With a Fluorinated Carbohydrate Moiety. Front. Plant Sci. 11:599228. doi: 10.3389/fpls.2020.599228
Received: 26 August 2020; Accepted: 16 November 2020;
Published: 10 December 2020.
Edited by:
Wolfram G. Brenner, Universität Leipzig, GermanyReviewed by:
Aaron M. Rashotte, Auburn University, United StatesSibu Simon, Central European Institute of Technology (CEITEC), Czechia
Copyright © 2020 Bryksová, Hybenová, Hernándiz, Novák, Pěnčík, Spíchal, De Diego and Doležal. This is an open-access article distributed under the terms of the Creative Commons Attribution License (CC BY). The use, distribution or reproduction in other forums is permitted, provided the original author(s) and the copyright owner(s) are credited and that the original publication in this journal is cited, in accordance with accepted academic practice. No use, distribution or reproduction is permitted which does not comply with these terms.
*Correspondence: Nuria De Diego, nuria.de@upol.cz